Take-Home Points
-
1. Dopamine turns on downstream second messengers when it acts at D1-like receptors and turns them off by acting at D2-like receptors.
-
2. Dopamine turns off its own release by its actions at presynaptic D3 or D2 autoreceptors.
-
3. D3 receptors are sensitive enough to detect dopamine that diffuses outside of the synapse, and can thus regulate the release of other neurotransmitters as well.
-
4. Dopamine can bind the family of dopamine receptors relatively selectively based simply on how much dopamine is released (lowest concentrations activate D3 receptors; moderate dopamine levels, D2 receptors; and high dopamine levels, D1 receptors). Dopamine can also differentially regulate postsynaptic neurons depending on the pattern of its release, ie, whether dopamine fires from its neuron tonically (baseline activity) or phasically (in bursts).
-
5. Dopamine receptor subtypes are distributed in different densities in specific brain areas, so dopamine can regulate its downstream neuronal circuits quite differently in various brain regions.
Introduction
Dopamine has distinct actions at each of its receptors, resulting in a wide range of regulatory functions.Reference Beaulieu and Gainetdinov 1 , Reference Stahl 2 Disruption of these actions by CNS disorders can lead to numerous psychiatric and neurologic symptoms.Reference Stahl 2 – Reference Nestler and Carlezon 6 Also, a plethora of psychotropic drugs can act upon dopamine receptors to ameliorate these symptoms.Reference Stahl 2 How can dopamine, which is only one neurotransmitter, have such widespread involvement in so many different brain disorders and with so many drugs? Understanding how dopamine normally functions in various regions of the brain can provide insight into the mechanisms underlying psychiatric and neurologic disorders and how they disrupt dopamine neurotransmission. This can also help us to see how various drugs may target dopamine neurotransmission to treat these disorders.
Dopamine Receptors
There are 5 major dopamine receptors divided into 2 classes: D1-like (D1 and D5 receptors) and D2-like (D2, D3, and D4 receptors)Reference Beaulieu and Gainetdinov 1 , Reference Stahl 2 (Figures 1A and 1B). Numerous dopamine agonists for Parkinson’s disease and antipsychotics for schizophrenia target one or more of these receptors.Reference Stahl 2 The presynaptic dopamine transporter (DAT), also called the dopamine reuptake pump, is a transmembrane protein (Figure 1A), as is the pump for transporting dopamine into synaptic vesicles, called vesicular monoamine transporter type 2 (VMAT2)Reference Stahl 2 (Figures 1A and 1B). Numerous psychotropic medications target either DAT or VMAT2 function; for example, stimulant medications aimed at treating attention deficit hyperactivity disorder (ADHD) target DAT, while new inhibitors for tardive dyskinesia target VMAT2.Reference Stahl 2 , Reference O’Brien, Jimenez and Hauser 7 Dopamine receptors are also therapeutic targets, and here we will focus on some potentially clinically relevant differences among D3, D2, and D1 receptors; their hypothetical links to CNS functions; and what happens when drugs target these receptors.
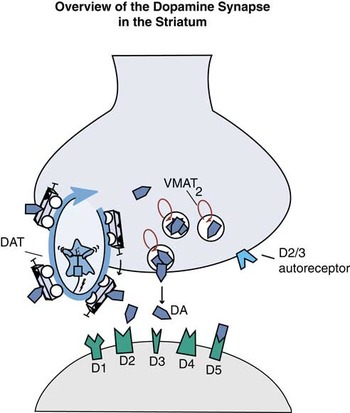
Figure 1A Overview of the dopamine synapse in the striatum. In the striatum, dopamine synapses are populated with presynaptic reuptake pumps called dopamine transporters (DAT), as well as presynaptic D2 or D3 autoreceptors, and presynaptic transporters of dopamine into synaptic vesicles called vesicular monoamine transporters type 2 or VMAT2. Postsynaptically, all 5 types of dopamine receptors can be present, including those that may be also located presynaptically, D2 and D3.

Figure 1B Overview of the dopamine synapse in the prefrontal cortex. In the prefrontal cortex, there is a relative paucity of DATs and D2/D3 receptors, so most of the action is upon postsynaptic D1 receptors. Lack of DATs and autoreceptors means that dopamine is free to diffuse away from the synapse after it is released, unlike in striatum (Figure 1A).
Second Messenger Systems and Differential Functions of Various Dopamine Receptors
Dopamine can have opposite effects on the same second messenger systems depending on the receptor subtype. That is, D1 receptors stimulate second messenger systems (Figure 2A), while D2 and D3 receptors inhibit themReference Beaulieu and Gainetdinov 1 , Reference Shen, Flajolet, Greengard and Surmeier 8 , Reference Tritsch and Sabatini 9 (Figure 2B). This plays an important role, for example, in motor control by the dorsal striatum, where D1 serves as a “go” signal and D2 serves as a “stop” signal.Reference Surmeier, Ding, Day, Wang and Shen 10 Loss of dopamine in this system leads to Parkinson’s disease; blockade of D2 receptors here causes short-term drug-induced extrapyramidal reactions and long-term tardive dyskinesia.Reference Stahl 2 . Levodopa stimulates both D1 and D2 receptors in the dorsal striatum, which initially restores motor function in Parkinson’s disease. Chronic use of levodopa, however, leads to structural changes in the striatum that cause maladaptive dyskinetic states.Reference Cenci and Konradi 11

Figure 2A Postsynaptic dopamine receptors either promote or inhibit second messenger systems. Shown here is how D1-like postsynaptic receptors promote second messenger systems. Specifically shown is G-protein–coupled stimulation of adenylate cyclase (AC) and cyclic adenosine monophosphate (cAMP) pathways.

Figure 2B Shown here is how D2-like post synaptic receptors inhibit second messenger systems. Specially shown is G-protein–coupled inhibition of adenylate cyclase (AC) and cyclic adenosine monophosphate (cAMP) pathways.
An alternative striatal circuit involving the nucleus accumbens (ventral striatum) is also heavily innervated with dopamine receptors, not only D1 and D2, but also D3, receptors. Dopamine receptors here, however, serve much different functions compared to those they mediate in the dorsal striatum (discussed below). Regardless of region, it is clear that D1, D2, and D3 receptors serve highly specific yet differential functions when bound by dopamine, allowing for the same neurotransmitter to regulate many different physiological, behavioral, and pathological states.
Regional Effects of Dopamine
In the ventral striatum, overactive dopamine release at D2 postsynaptic receptors hypothetically causes the positive symptoms of psychosis. Most antipsychotics target these D2 receptors to mitigate the effects of psychosis.Reference Stahl 2 D3 receptors are especially present in limbic areas, the hypothalamus, and the ventral tegmental area/substantia nigra, where they may regulate cognition, mood, and motivation.Reference Volkow and Baler 3 – Reference Nestler and Carlezon 6 , Reference Leggio, Bucolo, Platania, Salomone and Drago 12 – Reference Khan, Gutierrez, Martin, Penafiel, Rivera, De La and Calle 15 Dysregulation of the D3 receptor may result in a number of psychiatric disorders, especially depression and schizophrenia.Reference Leggio, Bucolo, Platania, Salomone and Drago 12 – Reference Khan, Gutierrez, Martin, Penafiel, Rivera, De La and Calle 15 Some antipsychotics can more potently target D3 receptors than D2 receptors,Reference Kiss, Horvath and Nemethy 16 with potentially favorable effects on mood, cognition, and negative symptoms.
In the prefrontal cortex, there is a low abundance of D2 and D3 receptorsReference Khan, Gutierrez, Martin, Penafiel, Rivera, De La and Calle 15 (Figure 1B), so most dopamine neurotransmission here is controlled by postsynaptic D1 receptors. When these D1 receptors are “out of tune” and either understimulated or overstimulated, this can hypothetically cause dysregulated mood, cognitive impairment, and negative symptoms (Figure 3). Many antipsychotics block D1 receptors as well as D2 receptors; this D1 receptor blockade (Figure 3) could theoretically reduce optimum dopamine stimulation and thereby contribute to cognitive and mood dysfunction by making neurotransmission at cortical synapses “out of tune.” Similarly, presynaptic D3 antagonists could theoretically cause disinhibition of dopamine release and “tune” cortical circuits to improve cognition, mood, and negative symptoms (Figure 3).Reference Leggio, Bucolo, Platania, Salomone and Drago 12 – Reference Kiss, Horvath and Nemethy 16

Figure 3 Functional outcome of cortical dopamine. For optimum dopamine activity in the prefrontal cortex, its release should be neither too high nor too low at its postsynaptic D1 receptors there. Thus, dopamine “tunes” its activity for best functioning, and this is hypothetically related to cognitive, mood, motivation, and other symptoms. If D1 receptors are blocked, for example by certain antipsychotics, this may cause dopamine at D1 receptors to be “out of tune” and produce cognitive symptoms. On the other hand, if D3 autoreceptors are blocked by other antipsychotics, this may cause more dopamine to be released and enhance theoretically reduced levels of dopamine in patients with cognitive symptoms, thereby improving these symptoms.
Autoreceptors
Both D2 and D3 receptors can be presynaptic as well as postsynaptic (Figures 1A, 1B, 4A, and 4B). When they are presynaptic, they can bind dopamine that is released from the presynaptic neuron, and through negative feedback mechanisms, prevent further release of dopamine. Synapses with presynaptic D3 autoreceptors may be turned off by lower levels of dopamine release into those synapses (Figure 4B) compared with synapses having presynaptic D2 autoreceptors (Figure 4A), since D3 receptors are much more sensitive to dopamine than are D2 receptors (Figure 5). D2 and D3 antagonists that block these autoreceptors disinhibit dopamine release but simultaneously would block the same receptors if present postsynaptically, creating a complex network of dopamine regulation both within and between brain regions.

Figure 4A Regulation of dopamine levels at the synapse. In synapses with D2 presynaptic autoreceptors, dopamine release may be relatively high because these receptors are relatively less sensitive to dopamine, allowing it to build up in the synapse.

Figure 4B In synapses with D3 presynaptic autoreceptors, dopamine release may be relatively more controlled because these receptors are most sensitive do dopamine, not allowing it to build up as much in the synapse.

Figure 5 Dopamine receptor affinities. The 5 dopamine receptor subtypes have differing affinities for dopamine, with the highest affinity being at D3 receptors; intermediate affinities at D2, D4, and D5 receptors; and lowest affinity at D1 receptors.
Interestingly, there appear to be few D2 or D3 receptors in prefrontal cortex (Figure 1B), and the few that are there may be localized on GABA interneurons and not at dopamine synapses.Reference Tritsch and Sabatini 9 This means that dopamine release could be much less tightly regulated in the cortex (Figure 1B) than in the striatum, where there are both D2 and D3 autoreceptors, for example (Figure 1A). This configuration combined with a relative lack of DATs to recapture synaptic dopamine in prefrontal cortex could enable dopamine to diffuse away from its synapse in the prefrontal cortex to allow nonsynaptic (volume) neurotransmission to occur (Figures 6 and 7B). Since D3 receptors sit on GABA interneurons, which innervate glutamate neurons, dopamine neurons, and other GABA neurons,Reference Tritsch and Sabatini 9 they are ideally located to regulate downstream glutamate, GABA, and dopamine neurotransmission.

Figure 6 Volume neurotransmission. Not all neurotransmission is synaptic (shown as number 1). In brain regions where dopamine can diffuse away from the synapse, such as the prefrontal cortex, which has a relative lack of presynaptic autoreceptors and dopamine transporters, a second kind of nonsynaptic neurotransmission can occur, called volume neurotransmission (numbers 2 and 3). Volume neurotransmission can occur if the diffusing dopamine reaches a dopamine receptor sensitive enough to bind it at these lower levels, such as the D3 receptor.
Volume (nonsynaptic) neurotransmission
Volume neurotransmission occurs without a synapse when a neurotransmitter diffuses to a nearby receptor that can detect it (Figure 6). D3 receptors are sensitive enough to detect dopamine when this neurotransmitter diffuses out of the synapse. In areas where dopamine levels are tightly controlled, such as in the striatum where dopamine synapses have presynaptic autoreceptors and where the DAT inactivating mechanism is present (see Figure 1A), very little dopamine may escape the synapse. However, in the prefrontal cortex, where there are very few DATs and very few D2 or D3 receptors (Figure 1B), dopamine released at the synapse is free to diffuse to other brain areas where dopamine receptors are present (Figure 6). This may be particularly relevant to extrasynaptic D3 receptors, which are both sensitive enough to detect dopamine diffusing out of synapses, and are also localized on other neurons, such as GABA neurons. Presynaptic D3 receptors on nondopamine neurons serve as heteroreceptors there, acting to inhibit the release of that neuron’s neurotransmitter, as mentioned above.
Synaptic neurotransmission could be thought of as analogous to “hard-wired” land lines for a telephone (Figures 1, 2, 4, and 7), whereas nonsynaptic (volume) neurotransmission can be conceptualized as being analogous to cell phones that transmit within the detection radius of a cell tower (Figure 6). Volume neurotransmission may be particularly important for cognitive functioning in the prefrontal cortex (Figures 1B, 3, and 6), diffusing widely to engage dopamine receptors.Reference Stahl 2 Dopamine thus acts very differently in prefrontal cortex (Figure 1B) than it does in the striatum (Figure 1A). DAT inhibitors and D2/D3 antagonists will not have robust actions in the prefrontal cortex because these receptors are not present in abundance there, but these same drugs do target DAT, D2, and D3 receptors in the striatum/nucleus accumbens and substantia nigra/ventral tegmental area, where these targets are indeed abundant.Reference Stahl 2 This helps to explain regional selectivity, not only of dopamine actions, but also the functional activity of drugs that target various dopamine receptors differently in different parts of the brain.
Dopamine Release
Dopamine can neuro-transmit at its associated receptors relatively selectively based simply on how much of it is released at the synapse, with the lowest concentrations activating D3 receptors, moderate concentrations activating D2 receptors, and high concentrations activating D1 receptors) (Figures 5 and 7).Reference Sunahara, Guan and O’Dowd 17 – Reference Van Tol, Bunzow and Guan 19 Dopamine can even differentially regulate postsynaptic neurons depending on whether dopamine fires from its neuron tonically (baseline firing) (Figure 7A) or phasically (in bursts) (Figure 7B).Reference Stahl 2 Tonic firing may set the “tone” of the synapse, and may also “tune” it (Figure 7A) so that it is ready for a barrage of phasic firing that can occur during “salient” interesting or rewarding stimuli, and thus shape both immediate and long-term behavior (Figure 7B).Reference Stahl 2 , Reference Volkow and Baler 3 , Reference Grace 20 This may be analogous to playing a guitar, where the tonic firing tunes the strings and phasic firing strums them, both of which must function correctly for the best output. Disruption of either tonic or phasic firing may lead to malfunctioning dopamine systems, and result in numerous behavioral problems ranging from psychosis to depression, addiction, and binge eating.Reference Volkow and Baler 3 , Reference Nestler and Carlezon 6 This may help explain why agents acting upon certain dopamine receptor targets in the specific malfunctioning part of the brain may result in therapeutic actions in a wide range of neurologic and psychiatric disorders.
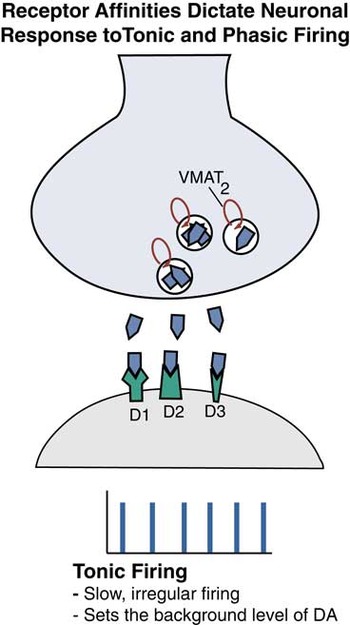
Figure 7A Receptor affinities dictate neuronal response to tonic and phasic firing. Tonic firing shown here is periodic, but slow and irregular, and is thought to set the background level of “tone” by dopamine (DA) at its synapses.
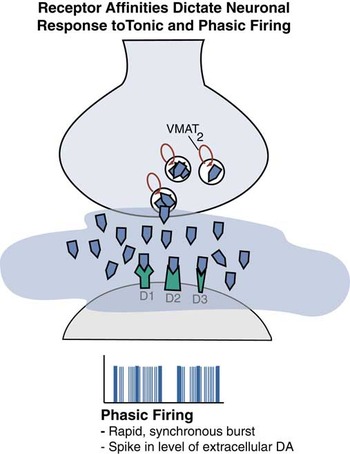
Figure 7B Phasic firing is shown here. This is a synchronous burst of spikes leading to a higher level of dopamine and a much different regulatory signal at the dopamine synapse compared to tonic firing.