Insomnia is the most common sleep disorder presenting as either difficulty falling asleep, maintaining sleep, or waking up early with inability to go back to sleep. 1 Insomnia affects 10–30% of the population. Insomnia and sleep deprivation are linked to a range of systemic medical conditions including dementia, depression, cardiovascular disease, and malignancy.Reference Castro, Poyares, Leger, Bittencourt and Tufik 2
Currently, medications are the mainstay of insomnia treatment, along with cognitive behavioral therapy for insomnia (CBT-I). Medications include unregulated supplements, antihistamines, off-label use of benzodiazepines, serotonin reuptake inhibitors (SSRIs), tricyclic antidepressants, and a few medications approved by the Food and Drug Administration (FDA). Further, hypnotics that have been approved for long-term use by the FDA are limited to two benzodiazepine agonists eszopiclone and slow-release zolpidem; selective melatonin receptor agonist, ramelteon; selective histamine H1 antagonist doxepin hydrochloride at low dose; and dual orexin (hypocretin) receptor antagonist, suvorexant. There is increasing tendency to limit long-term use of hypnotics either for the risk of tolerance development or other side effects.Reference Sateia, Buysse, Krystal, Neubauer and Heald 3
Brain stimulation has become an established treatment for multiple neurological disorders; however, its potential therapeutic effects in sleep medicine have not been fully explored. Seno et al. reported the effectiveness of rectangular pulses (1–3 V, 0.5 ms) for 187 s at gradually decreasing frequencies from 14 to 0 Hz.Reference Seno, Morita, Ikehata, Ishikawa, Nakayama and Matsumoto 4 In rodent models, low-intensity slow oscillating fields of 1 Hz frequency have been shown to induce similar 1 Hz delta slow cortical responses.5 Marshall et al. applied slow oscillating potentials at 0.75 Hz to healthy volunteers to show the effect of sleep on hippocampus-dependent declarative memory consolidation as well as sustaining the naturally occurring delta slow waves during non-rapid eye movement sleep.Reference Marshall, Helgadóttir, Mölle, Born and Marshall 6 Similarly, acoustic stimulation has been reported to augment slow-wave activity in both young and older adults and improve sleep-dependent memory consolidation.Reference Papalambros, Santostasi, Malkani, Braun, Weintraub, Paller and Zee 7
The purpose of this pilot prospective randomized single-blind crossover study was to investigate if augmenting theta-delta slow brain activity with low-intensity, low-frequency transcranial stimulation can induce and sustain N1-N3 sleep. Thirty adult patients with chronic insomnia who had failed multiple medications were evaluated. 1 Those with pregnancy, severe mood disorder, history of alcoholism and drug abuse, sleep apnea, and cardiopulmonary diseases were excluded. A total of nine patients, eight female, and one male (age 50 ± 10, mean 50.2) with chronic insomnia disorder (duration 4–13 years, mean 7.8 ± 3.4) completed the study and were included in the final analysis (Supplementary consort chart). The patients were not given instructions regarding sleep hygiene or CBT-I until the end of the study. A battery of standardized questionnaires was completed at the first visit and again at the end of the study 6 weeks later. Any hypnotics and SSRIs that had been prescribed merely for insomnia were tapered off over the initial 2 weeks so that by the time of their first polysomnograms (PSG), 14 d after the first visit, the patients were off medication and remained so for the following 6 weeks. All patients had two in-laboratory PSG, 2 weeks apart, during one of which they received tACS and the other night used as control (sham). The order of stimulation night vs sham was randomized; therefore, some patients were stimulated during their first PSG and others during their second PSG. The first PSG was done two weeks after the first clinic visit and the second study, two weeks after the first one. Sleep diaries were reviewed biweekly. All patients had a second/last clinic visit two weeks after the second PSG during which the above test battery was repeated (Figure 1). Informed consent was obtained from all patients. Ethical approval was granted by the institutional review board.
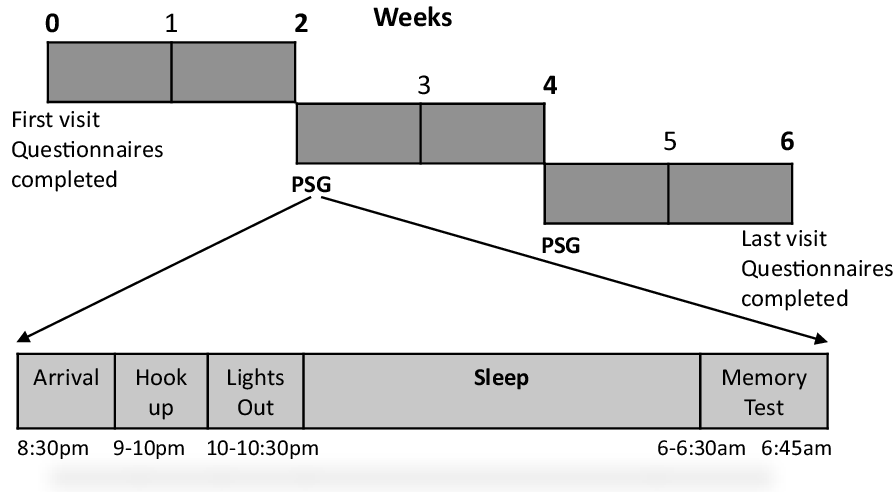
Figure 1: Diagram showing the sequence of clinic visits and timing of the two sleep studies.
Sleep data acquisition and manual scoring were done according to the American Academy of Sleep Medicine guidelines. Sleep onset latency (SOL), wake after sleep onset (WASO), arousal index, sleep efficiency, apnea/hypopnea index were recorded for analysis. Transcranial stimulation, adopted from Marshall et al.Reference Marshall, Helgadóttir, Mölle, Born and Marshall 6 , was applied as oscillatory sine wave currents delivered via the low noise “1X1 tES” device (Soterix Medical, New York, USA) at peak current intensity of 0.75 mA cm-2 and 0.75 Hz frequency providing a subthreshold charge density; therefore, the patients remained blind to the stimulation vs. sham. A 2X2 splitter and long cables were used for the remote triggering option. During both studies, two pairs of high-definition Ag-AgCl stimulation ring electrodes were placed bilaterally at F3 and F4 (frontal) and M1 and M2 (mastoid) positions to include major sleep-related structures in the current path. The electrodes were attached using regular Ten20 conductive paste and were kept in place via a special 4 mm thick plastic holder made by the manufacturer, and by wrapping; the patients did not report any focal pressure or discomfort related to the electrodes, and stimulation did not result in any skin lesions.
On stimulation nights, after lights out the subjects were stimulated for 5 min followed by a 1-minute pause to determine if the subject had entered sleep in which case no further stimulation would be applied. Given the stimulation artifact, those periods were scored as awake. If the patient remained awake for more than one minute after stimulation, another 5-minute stimulation would be applied, and this cycle would be repeated until sleep was achieved. In case of an arousal longer than 1 min, the above stimulation paradigm would be applied again. All stimulations were applied remotely and manually by the principal investigator (GKM). The patients did not feel the stimulation and remained blind to the intervention. During the sham studies, the same electrodes were placed, and the patients were hooked up to the tACS device but no stimulation was applied (Figure 2).
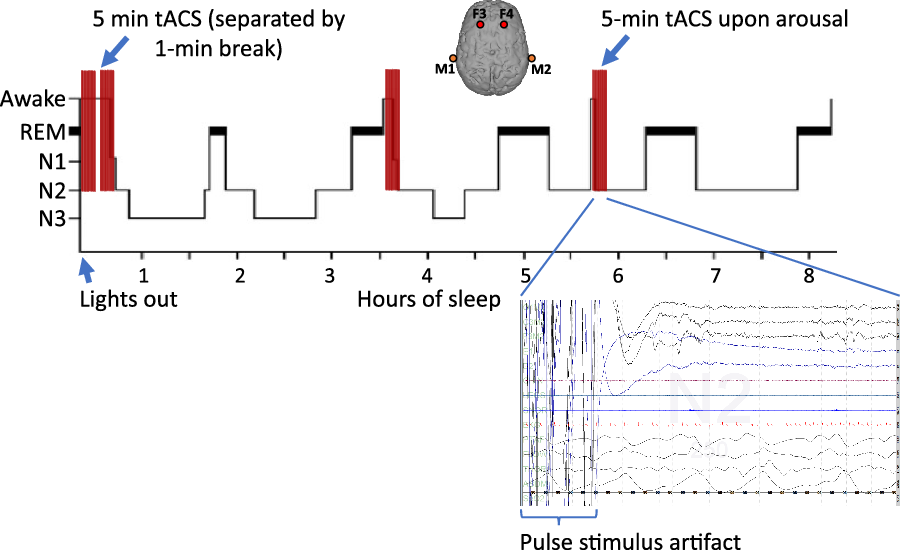
Figure 2: Stimulation paradigm. For consistency, the positive (anodal) electrodes were placed at F3 and F4 positions referenced to the negative (cathodal) electrode in the mastoids, bilaterally. Transcranial alternating current stimulation (tACS) started upon lights out as 5-minute sessions and in case the patient had not entered sleep, as checked during a 1-minute window, another 5-minute stimulation session would be applied; this cycle would be repeated until a sleep stage was observed on the EEG. The same paradigm would be applied again in case of an arousal lasting longer than 1 min. PSG recording at the end of a 5-minute stimulation session resulting in N2 sleep is shown. During the stimulation, the PSG signal recording is obscured by the stimulus artifact (30 s trace).
Each subject received a total of 10.2 ± 5.7 trials of stimulation of which 4.6 ± 2.4 (%45) induced sleep. The mean number of stimuli needed to induce the initial sleep period was 3.1 ± 3.6. Sleep onset latency dropped from 39.9 ± 56.3 min during sham study to 22.2 ± 25.8 min during the stimulation night, whether given on the first or second night, as well as improvements in sleep efficiency and WASO, but these differences did not reach statistical significance. However, there were significant drops in spontaneous arousals (8.6 ± 3 vs 7.1 ± 2.6, p = 0.04) and total arousals (16.3 ± 4.4 vs 14 ± 3.5, p = 0.02) (Table 1) (Supplementary Figure 1A–B). Given the small sample size and multiple outcome measures, the effect sizes were calculated for significant differences (Supplementary Table 1 with effect size). There were also significant improvements in multiple measures of long-term effects of stimulation as measured by the questionnaires and sleep diary. These included improvements in sleep quality (Pittsburg sleep quality index (PSQI) 14.4 ± 2.9 vs 8.2 ± 4.3, p = 0.001), sleep duration (5.6 ± 0.8 vs 6.3 ± 1.2 h, p = 0.04), SOL (62 ± 40 vs 37 ± 31 min, p = 0.005), WASO (85.7 ± 63.6 vs 44.7 ± 22.1 min, p = 0.05), sleep efficiency (69.8 ± 10.3 vs 82.2 ± 7.4%, p = 0.005), and Epworth sleepiness scale (7.6 ± 5.7 vs 6.7 ± 5.6, p = 0.09). There were no significant changes in measures of depression and anxiety (Table 2) (Supplementary Figure 1C).
Table 1: In-laboratory polysomnogram findings: stimulation vs sham

Entries are based on the mixed model and t-test results provided in the Stata Output for Lab data.
* Statistically significant difference. SOL = sleep onset latency; WASO = wake after sleep onset; min. = minute; Resp. = respiratory; Spon. = spontaneous; N1–3 = stages N1–3 sleep; REM = rapid eye movement (sleep); O2 sat. = oxygen saturation; AHI = apnea/hypopnea index; PLMI = periodic limb movement index; ESS = Epworth sleepiness scale.
Table 2: Standardized sleep-related questionnaires
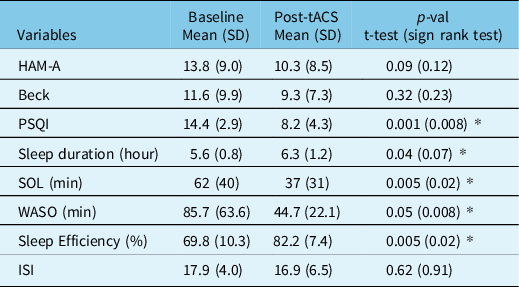
* Statistically significant difference. HAM-A = Hamilton anxiety rating scale; Beck = Beck’s Depression Inventory; PSQI = Pittsburg sleep quality index; SOL = sleep onset latency; WASO = wake after sleep onset; ISI = Insomnia Severity Index.
The quality of life (QOL) based on the SF-36 score showed significant improvements in multiple domains and the overall QOL (p = 0.02) (Supplementary Table 2, Figure 1D). The memory test showed significant improvement in recall after the second PSG (p = 0.04) (Supplementary Table 3; Figure 1E).
Transcranial current and auditory stimulations have been shown to increase the amplitude of slow oscillations, hence deepening sleep rather than improving sleep onset latency.Reference Marshall, Helgadóttir, Mölle, Born and Marshall 6 , Reference Papalambros, Santostasi, Malkani, Braun, Weintraub, Paller and Zee 7 Unlike those studies, we did not time-lock stimulation to the slow waves but instead started it during wakefulness. Therefore, the absence of significant effects on sleep initiation might suggest principal differences in mechanism of action of stimulation on different stages of sleep along with differences in mechanisms of sleep initiation vs slow sleep initiation/maintenance. Spontaneous sleep is initiated by cyclical dominance of the GABAergic ventrolateral preoptic area in the anterior hypothalamus over the wake-promoting monoaminergic nuclei of the brainstem. Therefore, stimulation of this structure, that was placed in the path of the current, might have contributed to the sleep-inducing effect of tACS. Further, we chose the site of stimulation based on the notion that including other critical structures, that is, thalamus, prefrontal cortex, and upper brain stem, in the path of current is critical to affect sleep generating networks, as reported previously. Among the possible mechanisms by which tACS may affect sleep is frequency-specific entrainment, that is, phase alignment of endogenous brain oscillations to the oscillating tACS currents.Reference Marshall, Helgadóttir, Mölle, Born and Marshall 6 Therefore, further experiments to optimize the stimulation paradigm are warranted. D’Atri et al. successfully induced sleepiness using bilateral tACS at 5 Hz but the subjects were all healthy volunteers.Reference D’Atri, Romano and Gorgoni 8 More recently, Wang et al. tried tACS in patients with insomnia as 40-minute stimulation sessions at much higher intensity and frequency for 20 d. The PSQI at week 8 showed a significant decrease along with improvements in several subjective sleep-related parameters. This study did not use overnight stimulation or PSG monitoring and was limited to subjective data acquisition.Reference Wang, Wang and Zhang 9
The main findings of our study include reduced arousal, improved sleep maintenance, more pronounced delayed effects, and absence of any immediate side effects of overnight application of transcranial electrical stimulation. Our stimulation paradigm did not show an immediate effect on SOL and WASO which may be attributed to a variety of factors including stimulation intensity and location as well as the small sample size. We avoided higher intensities to keep the patients blind to stimulation vs sham. It is conceivable that a higher charge density would have stronger therapeutic effects. The inter-subject variability in the number of stimuli to induce sleep may be attributed to inherent neurophysiological differences or severity of insomnia. Also, rebound insomnia might have affected the results since we tapered off hypnotics and SSRIs that had been prescribed for insomnia treatment. Given the small sample size of this pilot study, there was no significant association between outcome and the baseline medications. To our knowledge, this study is the first attempt at using overnight alternating current under PSG monitoring to acquire both objective and subjective measures in patients with chronic insomnia. We used tACS instead of tDCS to apply oscillatory currents similar to the naturally occurring delta slow waves generated in non-rapid eye movement sleep.
While insomnia is more common in females, it may not explain the significant predominance of females in our patient population which is a limitation of this pilot study. Another shortcoming might be the fact that we did not determine individual patients’ sensory thresholds to maximize the charge density according to each subject’s threshold. Further limitation is that outcomes from small cohorts cannot be generalized. Given the limitations of currently available treatments for insomnia in particular long-term use of hypnoticsReference Sateia 10 , it is pertinent to further investigate optimized tACS stimulation parameters.
Supplementary Material
To view supplementary material for this article, please visit https://doi.org/10.1017/cjn.2022.33
Acknowledgements
The authors are grateful to Kimford Meador, David Loring, Selima Rashid, April Moore, Diedre Durgapersad, Emily Paku, Faiza Adem, Ali Eyow, Nahshon Murray, and Jared Rice for their invaluable assistance throughout this study.
Funding
This work was supported by funding through the Partners in Research Award (grant 33,399), Georgetown University School of Medicine.
Conflict of Interest
Nothing to report.
Statement of Authorship
Conceptualization: Gholam K. Motamedi, Jian-young Wu, Peter E. Turkeltaub, Yong Won Cho. Data curation: Gholam K. Motamedi, Peter G. Jeliazkov. Formal analysis: Mihriye Mete, Gholam K. Motamedi, Shara Nawar. Investigation: all authors. Methodology: Gholam K. Motamedi, Jian-young Wu, Peter E. Turkeltaub. Writing – original draft: Gholam K. Motamedi. Writing – review & editing: all authors.