Introduction
Advancements in clinical decision-making, surgical techniques, and postoperative care have allowed spine surgeons to manage increasingly complex spine cases resulting in shorter surgery duration, reduced length of hospital stay, and improved clinical outcomes. Continued teamwork is required on the part of surgeons, scientists, allied professionals, and rehabilitation specialists to take this success forward. Among the various evolving practices in the field of spine surgery, this article will focus on one area that has shown considerable advancement during the past two decades: degenerative cervical myelopathy (DCM).
DCM is a progressive degenerative disease and the most common cause of nontraumatic cervical spinal cord injury. Reference David, Mohammadi and Martin1 The incidence of hospitalizations related to DCM has been estimated to be 4.04 per 100,000 person-years, and the number of patients undergoing surgical treatment each year has increased up to seven-fold. Reference Bakhsheshian, Mehta and Liu2 The rate and degree of neurologic deterioration is variable, and optimal management strategies, especially in cases with mild DCM, remain elusive. Given the limited regenerative potential of the spinal cord, early diagnosis and treatment of DCM are critical to reduce the risk of permanent disability. With changing demographics and an increasingly aging global population, DCM has become an important public health priority. Reference Sangondimath, Mallepally and Marathe3 The current article aims to provide a concise review of the latest advances and future directions in the management of DCM, with a special spotlight on “personalized approaches” to address the heterogeneity of the DCM patient population.
Causes of DCM
DCM represents an overarching clinicopathological entity encompassing a variety of age-related and genetically associated degenerative conditions of the cervical spinal column including degenerative disc disease (DDD), cervical spondylotic myelopathy (CSM), and ligamentous aberrations including ossification of the posterior longitudinal ligament (OPLL), (Figure 1). Reference Nouri, Tetreault and Singh4 On occasion, congenital disorders including Klippel-Feil Syndrome(KFS) and Ehlers-Danlos syndrome may contribute to the development of DCM. Reference Nouri, Tetreault and Singh4 These eventually culminate in chronic compression of the spinal cord causing neurological compromise through static and dynamic injury mechanisms. In this section, we will outline the clinical entities surrounding the term DCM as well as provide an overview on the pathophysiological mechanisms of chronic spinal cord compression encountered in DCM.
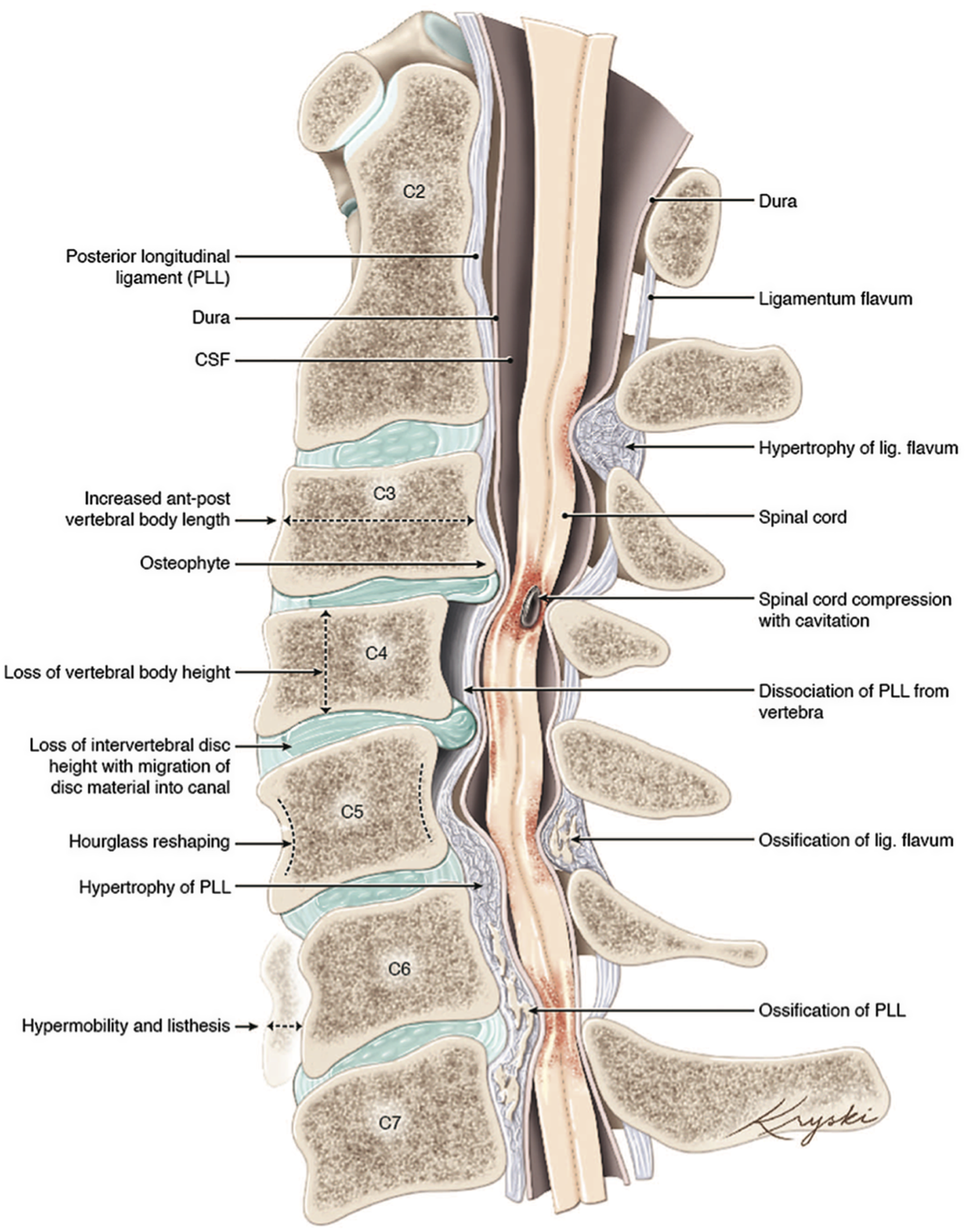
Figure 1: An illustration of the varied anatomical changes in the cervical spine that may underly the development of degenerative cervical myelopathy. Medical illustration by Diana Kryski (Kryski Biomedia). Reprinted with permission from Nouri A, Tetreault L, Singh A, et al. Degenerative Cervical Myelopathy: Epidemiology, Genetics, and Pathogenesis. Spine 2015;40:E675.
Degenerative Disc Disease (DDD) and Cervical Spondylotic Myelopathy (CSM)
The intervertebral discs (IVD) are composed of a collagen-rich outer ring called the anulus fibrosus and a proteoglycan-rich, hydrophilic and tumescent core, known as the nucleus pulposus. The IVD serves to bear axial compressive loads and to redistribute these forces along the vertebral endplates. Furthermore, owing to its viscoelastic nature, the nucleus pulposus provides a pivotal role in maintaining the IVD height. Reference Roberts, Evans and Trivedi5 Wear and tear due to aging, excessive mechanical stress, and/or environmental and nutritional factors cause the nucleus pulposus to degenerate and lose its viscoelastic properties. Reference Palepu, Kodigudla and Goel6,Reference Nixon7 As a result, the decreased IVD height alters the distribution of the axial forces leading to increased loads on the uncovertebral joints. Subsequently, the uncovertebral processes become flattened thereby increasing stress on the annulus and facet joints. Reference Baptiste and Fehlings8,Reference Kalsi-Ryan, Karadimas and Fehlings9 This ultimately initiates a cascade of degenerative changes. These changes comprise alterations to the disc (such as annular tears, disc bulging, or disc herniation), the formation of osteophytic spurs in an attempt to stabilize the abnormal motion and increase the surface of the weight-bearing endplates, and ligamentous hypertrophy or calcification. Reference Galbusera, van Rijsbergen and Ito10,Reference Badhiwala, Ahuja and Akbar11 Each individual degenerative change or a combination thereof contributes to the narrowing of the spinal canal and harbors the risk of spinal cord compression.
A static injury occurs through direct compressive forces or increased longitudinal stretching (caused by forcing the spinal cord over anterior bony spurs, protruded discs, or vertebral bodies in kyphotic deformities). Reference Ames, Blondel and Scheer12 In addition, dynamic mechanisms can cause movement-dependent worsening of compressions to the spinal cord through instability, increased range of motion and minor trauma in the setting of pre-existing DCM. Reference Nouri, Tetreault and Singh4 While neck flexion can potentiate the compressive effects of ventral osteophytes or disc herniations, hyperextension causes the ligamentum flavum (LF) to buckle and impinge the spinal cord posteriorly. Reference Tracy and Bartleson13,Reference Tetreault, Goldstein and Arnold14 On that note, the most common form of incomplete traumatic SCI, also known as central cord syndrome, usually occurs in older patients with pre-existing degenerative changes after low-impact hyperextension injuries to the cervical spine and is characterized by an asymmetric motor weakness, more profound in the upper than lower extremities. Reference Schneider, Cherry and Pantek15–Reference Ej, Mh and Gm17
Ligamentous Aberrations
Spinal degeneration may also result in structural changes to the posterior longitudinal ligament (PLL) and the LF, which include hypertrophy and aberrant ectopic ossification or calcification. These alterations ultimately result in a reduction of the spinal canal diameter and contribute to spinal cord compression. Reference Badhiwala, Ahuja and Akbar11
While the loss of IVD height has been discussed to cause abnormal cervical spine biomechanics that eventually lead to hypertrophic degeneration and buckling of the ligamentum flavum, Reference Baptiste and Fehlings8,Reference Kalsi-Ryan, Karadimas and Fehlings9 a nucleus pulposus prolapse has been proposed to be involved in the initiation of hypertrophic changes to the PLL. Reference Inamasu, Guiot and Sachs18 However, the exact underlying pathomechanism in the development of ossification of the PLL (OPLL), ossification of the LF (OLF), and calcification of the LF (CLF) remains unclear. While incidences of OPLL among Germans and North Americans have been reported to be 0.1 %, increased incidences of OPLL (1.9-4.3%), OLF (up to 20% in >65 years of age) and CLF among the Japanese population suggest environmental and/or genetic factors contribute to the marked calcification and ossification of these ligaments. Reference Baptiste and Fehlings8,Reference Matsunaga and Sakou19–Reference Matsunaga and Sakou21
Congenital Disorders
Several congenital disorders result in altered cervical spine anatomy and accelerate degeneration of cervical structures, thereby promoting earlier development and clinical manifestation of DCM.
Patients with Down syndrome (DS) potentially suffer from a variety of congenital manifestations, which, from a cervical spine perspective, include a predisposition to atlantoaxial instability (AAI), cervical spondylosis, and developmental abnormalities of bony structures (such as the odontoid process or the posterior arch of C1). Reference Ali, Al-Bustan and Al-Busairi22,Reference Bosma, Buchem and Voormolen23 The increased incidences of AAI among patients with DS have been numbered between 10–20%, of which however only 1–2% develop symptomatic myelopathy. Reference Ali, Al-Bustan and Al-Busairi22
Yet another hereditary disorder that renders the atlantoaxial joint at higher risk for instability is Ehlers-Danlos syndrome (EDS). Reference Henderson, Austin and Benzel24,Reference Halko, Cobb and Abeles25 EDS is a clinically and genetically heterogenous group of connective tissue disorders affecting mainly the skin, joints, and ligaments. Reference Steinmann, Royce and Superti-Furga26 The atlantoaxial joint is essentially dependent on the stabilizing properties of the transverse and alar ligaments, which in EDS show a proclivity to inefficiency thereby increasing the risk for AAI. Reference Henderson, Austin and Benzel24,Reference Halko, Cobb and Abeles25 Furthermore, ligamentous laxity puts patients with EDS at an increased risk of developing segmental instabilities and kypho-scoliotic deformities. Reference Henderson, Austin and Benzel24,Reference Steinmann, Royce and Superti-Furga26 As a result of a loss of physiologic cervical lordosis and increasing kyphosis, normal elongation of the spinal cord during neck flexion may worsen due to compression against the vertebral bodies and/or ventral spondylotic bars. Reference Henderson, Austin and Benzel24,Reference Shedid and Benzel27
KFS is a complex, congenital condition, which has been shown to present with a classical clinical triad of low posterior hairline, short neck and limited cervical range of motion in 34–74% of patients. Reference Samartzis, Herman and Lubicky28,Reference Tracy, Dormans and Kusumi29 Although various neurologic and visceral abnormalities have been shown to be associated with KFS, the hallmark of this condition is the improper segmentation of cervical motion segments, resulting in congenitally fused vertebrae. Reference Samartzis, Herman and Lubicky28,Reference Tracy, Dormans and Kusumi29 The abnormal vertebral fusion seen in KFS patients is mainly observed in the cervical spine and has been linked to facilitate similar degenerative processes as seen in patients who have undergone operative fusion of cervical motion segments. The effects, which have been discussed to occur as a result of increased biomechanical stress on adjacent nonfused segments, include the development of adjacent level disc degeneration, cervical spondylotic development, subluxation, bulging of the disc and/or LF, and loss of cervical alignment. Reference Samartzis, Herman and Lubicky28,Reference Eck, Humphreys and Lim30,Reference Goffin, Geusens and Vantomme31
Developmental narrowing of the cervical spinal canal, as seen in congenital spinal stenosis, has been shown to lower the threshold at which the cumulative effects of degenerative changes encroaching the spinal cord cause clinical signs and symptoms of DCM. Reference Nouri, Tetreault and Singh4,Reference Baptiste and Fehlings8,Reference Bernhardt, Hynes and Blume32,Reference Yue, Tan and Tan33 While normal sagittal canal diameters have been reported to be 17–18 mm between C3 and C7, a sagittal diameter of <13 mm constitutes a risk factor for developing signs and symptoms of cervical myelopathy. Reference Baptiste and Fehlings8,Reference Bajwa, Toy and Young34 Clearly, interindividual variabilities of what is considered a “normal” spinal canal diameter, variations in the thickness of the spinal cords, and imaging artifacts complicate the development of uniform thresholds when taking into account absolute values. The Torg-Pavlov method compares the spinal canal diameter with the corresponding vertebral body (spinal canal/vertebral body), whereby a ratio of 0.82 has been found to suggest significant cervical spinal stenosis. Reference Pavlov, Torg and Robie35
Pathophysiology of Spinal Cord Compression
The understanding of the pathophysiological mechanisms in DCM has long been complicated due to the lack of commensurable animal models capable of reflecting the effects of chronic compression to the spinal cord. The development of robust animal models resulted in meaningful comprehension of the underlying pathomechanisms, including ischemia, blood-spinal-cord barrier (BSCB) disruption, inflammation, and apoptosis. Reference Baptiste and Fehlings8,Reference Klironomos, Karadimas and Mavrakis36,Reference Karadimas, Moon and Yu37 Eventually, these events have been demonstrated to culminate in axonal demyelination and degeneration of corticospinal tracts, astroglial scarring, cavitation, loss of interneurons, as well as atrophy and degeneration of anterior horn cells. Reference Kalsi-Ryan, Karadimas and Fehlings9
The cascade of detrimental effects in DCM is essentially nourished as the spinal cord macro- and microvasculature experiences chronic compression, thereby causing ischemia through attenuation of local and regional spinal cord blood flow. Reference Karadimas, Gatzounis and Fehlings38 While the compressive effects exerted by degenerative changes understandably cause extraluminal narrowing of the major feeding arteries (such as the vertebral arteries and anterior spinal artery), pathological findings have identified vessel wall thickening and hyalinization to occur in the anterior spinal artery and parenchymal arterioles. Reference Brain, Knight and Bull39,Reference Mair and Druckman40 On a microvascular level, local perfusion is impaired as chronic cord compression causes stretching, flattening, and loss of penetrating branches of the lateral pial arterial plexus. Reference Kalsi-Ryan, Karadimas and Fehlings9,Reference Breig, Turnbull and Hassler41
Endothelial cells, which are conjoined with tight junctions, are of fundamental importance to maintain the integrity of the BSCB. Ischemia-induced hypoxic endothelial cell death and dysfunction renders the BSCB permeable, thereby increasing vasogenic spinal cord edema, influx of inflammatory cells, and entry of blood-borne toxins. Reference Kalsi-Ryan, Karadimas and Fehlings9,Reference Karadimas, Moon and Yu37,Reference Karadimas, Gatzounis and Fehlings38 In a rat CSM model, Karadimas et al demonstrated increased macrophage/microglia expression in conjunction with a disrupted BSCB, thereby providing evidence of neuroinflammation as a key injury mechanism involved in DCM. Reference Karadimas, Moon and Yu37
The subsequent neuroinflammatory process is characterized by recruitment of neutrophils, activated microglia, macrophages, and lymphocytes to the lesion site. Reference Yu, Liu and Kiehl42,Reference Hirai, Uchida and Nakajima43 One mechanism for how activation of microglia and recruitment of macrophages is being achieved has been identified in the CX3CL1/R1 signaling pathway. Reference Harrison, Jiang and Chen44 CX3CR1, a receptor highly expressed on microglia has been found to be increased in postmortem human cervical spinal cords from patients with CSM. Reference Yu, Liu and Kiehl42 The ligand CX3CL1 (fractalkine), which is usually bound on neuronal membranes, gets cleaved after neuronal injury and acts as a soluble chemokine. Reference Bazan, Bacon and Hardiman45,Reference Mizuno, Kawanokuchi and Numata46 This finding has prompted further animal studies, wherein CX3CR1-deficient knockout-mice showed decreased microglia/macrophages accumulation and inflammation at the site of injury resulting in increased neuronal and oligodendroglial preservation as well as improved functional neurological recovery. Reference Yu, Liu and Kiehl42 Neuronal and oligendroglial cell death are hence precipitated by the effects of neuroinflammation. Reference Yu, Baptiste and Liu47,Reference Karadimas, Gialeli and Klironomos48 These effects ultimately peak in the activation of the Fas-, Reference Yu, Liu and Kiehl42,Reference Yu, Baptiste and Liu47 tumor necrosis factor-alpha Reference Inukai, Uchida and Nakajima49,Reference Uchida, Nakajima and Watanabe50 and mitogen-activated protein kinase pathways-mediated apoptosis. Reference Takenouchi, Setoguchi and Yone51
Finally, although surgical decompression of the spinal cord aims at halting the progression of neurologic decline in DCM, 7-11% of patients show neurologic deterioration after surgery. Reference Fehlings, Badhiwala and Ahn52 One explanation underlying postoperative neurologic deterioration has been found in reperfusion-mediated oxidative stress. Restoration of tissue blood flow in a previously prolonged ischemic spinal cord-microenvironment has been shown to induce oxidative stress, resulting in damage to neurons in both human and rodents, a process also known as ischemia-reperfusion injury. Reference Karadimas, Laliberte and Tetreault53
Natural History of DCM
The natural history of DCM, particularly for individuals with mild impairment, remains incompletely understood. The heterogeneity of patient presentation and underlying genetic susceptibility to the effects of chronic cord compression adds to the challenges in defining the clinical presentation and course of DCM. Genetic factors, including the ApoE allelic status, have been shown to influence the natural history of DCM. Of note, patients with an ApoEϵ4 gene polymorphism and chronic cervical spinal cord compression were found to be at an increased risk of developing DCM and to have a comparatively poor natural history when compared to those with an ApoE3 genetic signature. Reference Setzer, Hermann and Seifert54
Rates of neurologic deterioration with nonoperative strategies have been estimated to range between 20–62% at 3–6 years of follow-up, as quantified by the modified Japanese Orthopedic Association scale (mJOA). Reference Karadimas, Erwin and Ely55 However, these numbers likely underestimate the actual rate of deterioration as it can be subtle and patients often fail to recognize worsening symptoms due to mechanisms of behavioral adaption and neuroplasticity. Reference Martin, De Leener and Cohen-Adad56,Reference Kalsi-Ryan, Clout and Rostami57 Notably, a recent ambispective cohort study from our group examined 117 patients (74 mild, 28 moderate, 15 severe DCM) and found a relatively high rate of neurological deterioration in 57% of primarily diagnosed DCM (95% CI, 46–67%) as well as 73% for recurrent DCM (95% CI, 50–88%) over a mean follow-up period of 2.5 years. Of concern, we found that the mJOA score and conventional structural MRI were relatively insensitive to detect clinical change. Reference Martin, Kalsi-Ryan and Akbar58 As a result, we would conclude that the mJOA score or anatomical MRI alone are not sufficient for clinical-radiologic monitoring of myelopathic progression. Serial clinical assessments should therefore include quantitative grip strength, dexterity testing, balance and gait analysis, with the aid of ancillary tests as further outlined below. Reference Kalsi-Ryan, Clout and Rostami57 This holds particularly true for patients with mild DCM in whom a nonoperative strategy might have been chosen initially, and where early detection of neurologic deterioration is of utmost importance to transition from a nonoperative approach to surgery.
And yet, while surgery has been shown to be safe and effective, Reference Badhiwala, Witiw and Nassiri59 further research on the natural history of mild DCM is required to aid in clinical decision-making for this subset of patients.
Diagnosis of DCM
The early diagnosis of DCM is essential as delays will result in irreversible nerve impairment for patients. Primary care providers (PCPs) are often the first to encounter a patient with a potential case of DCM. Unfortunately, the diagnosis of DCM in the primary care setting is often delayed. Reference Behrbalk, Salame and Gilad60 As a result, efforts to enhance the diagnosis of DCM and educate PCPs in the diagnosis of DCM are paramount.
Clinical Signs and Symptoms
The early clinical signs of DCM can be subtle. Common presenting symptoms include upper extremity paresthesias, sphincter dysfunction, gait disturbances, and fine motor dysfunction. The clinical signs of DCM are encompassed by the mJOA (Table 1). Reference Martin, De Leener and Cohen-Adad56 This scale can be used to assign a score to patients from their upper and lower extremity motor function, upper extremity sensory function and their sphincter function. Patients can then be stratified into mild, moderate, and severe disease based on their mJOA score. The physical exam signs of DCM are characterized by upper motor neuron signs (hyperreflexia, Babinski sign, Hoffman’s sign), focal motor deficits, sensory deficits, gait ataxia, and positive Romberg sign. A thorough exam and history taking is essential in the diagnosis and monitoring of DCM patients.
Table 1: The modified Japanese Orthopedic Association (mJOA) classification. The sum of the subscores from each of the four categories defines the severity of degenerative cervical myelopathy: mild (15–17); moderate (12–14); and severe (0–11) degenerative cervical myelopathy

The signs and symptoms of DCM are broad and can also be present in other neurological conditions. The differential diagnosis of DCM includes, radiculopathy, peripheral neuropathy, stroke, amyotrophic lateral sclerosis, diabetic neuropathy, Chiari malformation, and tumors. Patients with early DCM are often misdiagnosed with bilateral carpal tunnel given similar presentations. Whenever DCM is suspected, it is essential to carry out a thorough clinical and physical examination, as well as to obtain imaging and electrophysiological testing when appropriate. When the clinical diagnosis of DCM is uncertain, a referral to a neurologist or spinal surgeon is warranted.
Imaging Techniques
The diagnosis of DCM requires clinical signs and symptoms of cervical myelopathy and imaging evidence of cervical cord compression. Magnetic resonance imaging (MRI) is the imaging modality of choice for the diagnosis of DCM. In cases where an MRI cannot be obtained, CT myelography can be substituted. Patients with mild DCM can have subtle imaging findings. Any changes that cause the deformation of the cord can result in DCM. Cord signal change and circumferential compression of the cord are not required for the diagnosis of DCM. Reference Nouri, Martin and Mikulis61
The diagnosis of DCM can potentially be transformed with advancements in MRI modalities. There are emerging techniques that provide further granularity on the microstructural integrity of the spinal cord. One of these quantifying techniques is T2*-weighted imaging, which can provide a sensitive assessment of cord integrity and potentially be used in monitoring disease progression. Reference David, Mohammadi and Martin1,Reference Martin, De Leener and Cohen-Adad62 With T2*-weighted imaging, one can compare the signature ratio of the white and gray matter as a biomarker of white matter injury (T2*WI WM/GM). With these advancements in MRI modalities and the ability to quantify the structural integrity of the cord, we can expect a transition to a personalized approach to the imaging diagnosis and monitoring of DCM. However, further research is required prior to widespread utilization of these emerging techniques.
Ancillary Tests
As the care of DCM continues to evolve, there is a need for personalized clinical assessment and diagnostic tools. Reference Wilson, Badhiwala, Moghaddamjou, Martin and Fehlings63 Given the varied disease presentation, a single ancillary test is not feasible for the diagnosis of DCM. Specialized testing of cord function, including using the Timed Up and Go, Reference Richardson64 GAITRite, Reference Bilney, Morris and Webster65,Reference Van Uden and Besser66 and the Graded Redefined Assessment of Strength Sensibility and Prehension protocol (GRASSP), Reference Kalsi-Ryan, Curt and Verrier67 can be used to provide further granularity in the clinical assessment of patients with DCM. Adopting these personalized measurement tools requires a multidisciplinary approach to care with an emphasis on a personalized approach that will allow for enhanced discovery of disease progression. Reference Moghaddamjou, Wilson and Martin68
The Role of Biomarkers
Ongoing research in the field of DCM biomarkers will provide us with tools aimed at tailoring patient-specific therapeutic approaches. This becomes all the more important, as the disease is usually accompanied by a subtle progression of neurologic deterioration in a particularly aged patient population. Biomarkers hold the potential to increase the accuracy of diagnosis, provide us with a tool to monitor disease progression, offer prognostic information for patients and their relatives, as well as aid in clinical decision-making.
Recently, circulating microRNAs have emerged as potential serum biomarkers for SCIs. One such microRNA, termed MIR21-5p, has been found to play a key role in the regulation of neuroinflammation in DCM. Reference Laliberte, Karadimas and Vidal69 In human and rodent studies of DCM, MIR-21-5p upregulation has been shown to correlate with initial symptom severity and poor treatment outcomes, thereby providing a tool for outcome prediction. Reference Laliberte, Karadimas and Vidal69 Other micro RNAs, such as MIR-10a, MIR-210, and MIR-563, have been shown to be potentially useful in diagnosing OPLL. Reference Xu, Zhang and Zhou70 While the diagnosis of OPLL is particularly imaging-dependent, serum biomarkers might be useful as a fast and readily available, broadly applicable screening tool in high risk populations (i.e., Japanese >65 years of age) or where imaging is not available at hand.
Apolipoprotein E (APOE) has been suggested to play a critical role in repair and regeneration processes after lesions to the CNS with an impairment of this function in proteins with the isoform E4 coded by the ϵ4 allele. Reference Setzer, Hermann and Seifert54 The presence of an ApoEϵ4 gene polymorphism, as detected by DNA analysis from venous blood samples, has been associated with an increased risk of developing myelopathy in patients with chronic cervical spinal cord compression as well as a lack of neurologic improvement after surgical cord decompression. Reference Setzer, Hermann and Seifert54,Reference Setzer, Vrionis and Hermann71 A recent study investigated the effects of surgical decompression in a clinically relevant DCM model in genetically modified mice that possessed the human ApoE4 gene.(Article, PMID: 34369386) As compared to their ApoE3 counterparts, mice which were expressing human ApoE4 revealed delayed neurobehavioral recoveries post-decompression surgery. Notably, this was accompanied by an exacerbated pro-inflammatory response resulting in higher concentrations of TNF-α, IL-6, CCL3, and CXCL9. These exciting findings suggest that an at risk ApoE4-positive DCM patient population potentially benefits from personalized strategies in the treatment of DCM.
Ancillary technical tools, such as electrophysiology, have demonstrated promising results in improving diagnostic accuracy and providing prognostic information. Reference Jutzeler, Ulrich and Huber72,Reference Holly, Matz and Anderson73 As delineated above, imaging characteristics can be correlated to disease severity. Further technical advancements as well as the field of artificial intelligence and machine learning hold the potential to substantially increase the sensitivity and prognostic value of imaging studies in the near future.
Clinical Decision-Making
The main decision surrounding the management of DCM is surgical vs. nonoperative management. According to the AO Spine clinical practice guidelines, patients with severe (mJOA < 12) and moderate (mJOA 12–14) myelopathy should be offered surgery unless contraindicated. Reference Fehlings, Tetreault and Riew74 In patients with mild myelopathy without clinical progression, there is equipoise between operative managements and a structured supervised trial of conservative management.
Given the uncertainty in the management of mild DCM patients, a personalized approach to their care is required. A patient’s general health, co-morbidities, functional status, and occupational status can all be factored into the decision-making. Ultimately, a patient’s preference in management will often dictate their care. If a nonoperative approach to care is adopted, it is essential that patients are followed closely for clinical progression. Those patients with clinical progression should be re-considered for surgical intervention. Patients themselves are often not aware of disease progression, and early signs can be difficult to detect due to neuroplasticity, adaption and behavioral modifications. Reference Martin, De Leener and Cohen-Adad56 These patients should have frequent follow-up and a multidisciplinary team with personalized functional ancillary testing in their monitoring.
Guidelines for the Management of Patients with DCM
In 2017, a multidisciplinary guideline development group under the auspices of AO Spine North America and the Cervical Spine Research Society conducted five systematic reviews and developed recommendations for the management of DCM. Reference Fehlings, Tetreault and Riew74 The quality of evidence and the strength of recommendations were determined by the Grading of Recommendation, Assessment, Development and Evaluation (GRADE) guideline development tools. Reference Balshem, Helfand and Schünemann75–Reference Andrews, Schünemann and Oxman77 The results are portrayed in this section:
-
Patients with severe DCM (mJOA 0–11): Surgical intervention is recommended (Quality of evidence: moderate; Strength of recommendation: strong)
-
Patients with moderate DCM (mJOA 12–14): Surgical intervention is recommended (Quality of evidence: moderate; Strength of recommendation: strong)
-
Patients with mild DCM (mJOA 15–17): Offering surgical intervention or a supervised trial of structured rehabilitation is suggested. If nonoperative management is initially pursued, surgical intervention is recommended if neurologic deterioration occurs. A surgical intervention is suggested if patients fail to improve. (Quality of evidence: very low to low; Strength of recommendation: weak)
-
Nonmyelopathic cervical cord compression without signs or symptoms of radiculopathy: Offering prophylactic surgery for nonmyelopathic patients with evidence of cervical cord compression without signs or symptoms of radiculopathy is not suggested. It is suggested that these patients be counseled and educated as to potential risks of progression and about relevant signs and symptoms of myelopathy, and be followed clinically. (Quality of evidence: no evidence, clinical expert opinion; Strength of recommendation: weak)
-
Nonmyelopathic cervical cord compression with clinical evidence of radiculopathy with or without electrophysiological confirmation: Patients should be counseled about the higher risk of developing myelopathy. Surgical intervention or nonoperative treatment consisting of close serial follow-up or a supervised trial of structured rehabilitation is suggested. (Quality of evidence: low; Strength of recommendation: weak)
Operative Treatment
The ultimate goal of surgical intervention is to remove the compressive forces from the spinal cord thereby restoring spinal cord blood flow. Moreover, kyphotic deformities and/or spinal column instability may require instrumented fusion to restore cervical alignment or ensure mechanical stability. Historically, the target of operative decompression was to halt the progression of neurologic deterioration and to maintain the neurologic status. However, newer studies have shown improved long-term outcomes of neurologic function, disability, and health-related quality of life in patients receiving surgical decompression for DCM, therefore adding more weight to the indication for operative treatment. Reference Fehlings, Wilson and Kopjar78,Reference Fehlings, Ibrahim and Tetreault79 Moreover, a recent study has demonstrated that patients who were operated within four months of symptom onset had significantly better long-term mJOA outcomes than those treated after four months. Reference Tetreault, Wilson and Kotter80
Owing to the heterogeneity of the pathologies encountered in DCM, different surgical approaches and interventions have been developed to reach optimal decompression of the spinal cord. These include anterior approaches, such as cervical discectomy and fusion (ACDF), anterior cervical corpectomy and fusion (ACCF), a combination of ACDF and ACCF, cervical disc arthroplasty and posterior approaches, such as posterior laminectomy with or without posterior instrumented fusion or laminoplasty. A third category encompasses circumferential decompressive and stabilizing surgeries, which combine both anterior and posterior techniques and are usually confined to more complex and severe cases.
Anterior Versus Posterior Surgical Approach
While in younger patients with ventrally located, focal pathologies (such as single- or two-level DDD), an anterior surgical approach is usually favored, Reference Fehlings, Barry and Kopjar81 decision-making in other scenarios might not be as clear. The seemingly equipoise between anterior and posterior approaches in these cases has generated a source of vibrant debates about the optimal surgical approach. Reference Fehlings, Barry and Kopjar81–Reference Kato, Nouri and Wu83 A prospective observational multicenter study of 264 patients demonstrated no significant differences in rates of complications and functional or quality of life outcomes as measured by the mJOA, Nurick Scale, Neck disability index (NDI), and SF36v2 scores. Reference Fehlings, Barry and Kopjar81 These findings have been underscored by a propensity-matched analysis of a combined dataset of two large prospective multicenter AO Spine studies where anterior and posterior surgical decompression resulted in no significant differences in rates of complications and postoperative outcomes at 2 years. Reference Kato, Nouri and Wu83 A recent quality outcomes database analysis of 245 patients undergoing three to five-level surgical decompression for DCM (Anterior = 163, Posterior =82) strengthened the previous findings of similar patient reported outcomes and found no significant differences in readmission and return to work rates. Reference Asher, Devin and Kerezoudis82 Finally, the results of a North American multicenter RCT (n = 163; anterior surgery n = 63; dorsal surgery n = 100) have just recently been published confirming that an anterior surgical approach did not significantly improve patient-reported physical functioning at 1 or 2 years as indicated by the Physical Component Summary score of the SF-36v2. Reference Ghogawala, Terrin and Dunbar84 However, patients undergoing anterior surgery had a significantly greater risk of complications than dorsal surgery (47.6% vs 24%; 95% CI, 8.7–38.5%; P = 0.002). Of particular note, most of the complications were minor, including dysphagia (41% vs. 0% for anterior vs posterior surgery, respectively), and resolved within one year after surgery.
In sum, the current evidence does not show superiority of one surgical approach over another. And yet, in order to provide optimal surgical treatment and move toward an individual, patient-tailored approach, several factors need to be considered including the underlying pathology, management of sagittal deformity as well as surgical expertise. Reference Nouri, Martin and Nater85
Considerations in Surgical Decision-Making
Several clinical factors have been shown to affect outcomes from DCM surgery including patient age, duration of symptoms, comorbidities, preoperative imaging characteristics and the restoration of sagittal alignment. Reference Wilson, Jiang and Fehlings86
Indeed, recent studies have ascribed increasing importance to the consideration of preoperative cervical alignment parameters (such as the C2–7 angle and the modified K-line method) in surgical decision-making. Reference Shamji, Mohanty and Massicotte87 Cervical lordosis, for example, is commonly assessed from C2–C7 using the Cobb angle method. Reference Ames, Blondel and Scheer12 In that regard, patients with a cervical kyphosis exceeding 13° have demonstrated improved neurologic recoveries when undergoing an anterior surgical approach or posterior surgery where the correction of local kyphosis is being addressed by instrumentation as compared to laminoplasty. Reference Suda, Abumi and Ito88 Another helpful measurement tool attempting to predict residual anterior compression of the spinal cord after DCM surgery has been introduced with the modified-K line method. Reference Taniyama, Hirai and Yamada89 On a mid-sagittal cervical MRI, the modified K-line represents a connecting line from the sagittal midpoints of the spinal cord at the levels of C2 and C7. The minimum interval distance (mINT) describes the distance between the anterior compressing elements and the modified K-line. Using this concept, the authors suggest a mINT of >4.4 mm as a cutoff for an optimal neurologic recovery in nonlordotic patients after laminoplasty. Reference Taniyama, Hirai and Yoshii90 On the other hand, a mINT of <4 mm should direct the surgeon toward an anterior or combined anterior/posterior approach in order to avoid residual anterior compressive forces and achieve best possible neurologic outcomes. These data clearly stress the importance of cervical alignment parameters, which as a result should be incorporated into surgical decision-making.
Factors that guide the choice between the varied anterior or posterior surgical techniques have been recently reviewed in detail by Wilson et al. Reference Wilson, Tetreault and Kim91 In brief, when an anterior surgical approach is chosen in the setting of minimal retrovertebral disease, a multilevel ACDF is recommended over an ACCF or a hybrid ACDF-ACCF approach. However, in the presence of significant retrovertebral disease, a hybrid discectomy-corpectomy approach is preferred over multiple corpectomies. Reference Wilson, Tetreault and Kim91,Reference Shamji, Massicotte and Traynelis92
In the setting of a posterior approach, the current body of evidence does not show superiority of one surgical procedure over the other (laminoplasty versus laminectomy and fusion) in terms of neurologic outcomes. Reference Wilson, Tetreault and Kim91,Reference Fehlings, Santaguida and Tetreault93–Reference Yoon, Hashimoto and Raich96 This stresses the importance of having a high-quality RCT in order to provide final clarity on this subject.
Nonoperative Management
A structured nonoperative approach is often adopted in the management of patients with mild DCM. It is imperative that patients who are receiving nonoperative management are monitored as any clinical deterioration would change their candidacy for surgical intervention. There is variability in different approaches to nonoperative treatment and patient specific factors with available resources at the treating facility often dictating the approach to care. Non-operative protocols include immobilization, cervical bracing, neck therapy, cervical traction, and a combination of drug therapies. Reference Wilson, Tetreault and Kim91 Patients with a soft disc herniation and dynamic myelopathy can achieve resolution of their symptoms with structured neck therapy. Reference Fehlings, Tetreault and Riew74 Specific physiotherapy protocols have also been published demonstrating pain relief as well as functional improvements. Reference Spooren, Janssen-Potten and Kerckhofs97–Reference Binder100
Currently, medical, and regenerative therapies are in their infancies for the treatment of DCM. Halting the chemical cascade behind degeneration is an ideal target for medical therapies. Mesenchymal stem cells Reference Wang, Perez-Terzic and Smith101,Reference Urits, Capuco and Sharma102 and therapeutic protein injections Reference Masuda, Imai and Okuma103,Reference Wei, Williams and Bhargav104 have been studied and show early promising results in the treatment of DCM. Off label use of Riluzole has shown promising pre-clinical results in improving outcomes in DCM. Reference Karadimas, Laliberte and Tetreault105 In clinical trials, while the use of adjuvant Riluzole did not improve functional outcomes beyond surgical decompression, there were improvements in secondary outcomes such as pain which warrant further investigation. Reference Fehlings, Badhiwala and Ahn106 Further research could isolate populations that would benefit from adjuvant therapies such as Riluzole.
Illustrative Case
We present the case of a 62-year-old, right-handed female who was seen by her PCP due to neck pain. She was initially given a diagnosis of bilateral carpal tunnel syndrome when she presented with paresthesia in both hands. The patient was started on a trial of braces with no alleviation of her symptoms. On further history she indicated that she has been dropping objects. Suggestive of impairment of her manual dexterity, she could button up her shirt only with great difficulty. The patient could not go up the stairs without holding on to a handrail indicating potential gait imbalances. There was no history of trauma.
On physical examination, the patient had intact strength in all the major muscle groups of the lower and upper extremities. She had a positive Hoffman sign bilaterally. She showed brisk reflexes in the biceps and triceps tendons bilaterally. The patient had marked difficulty with tandem gait. Sensation was altered to light touch and pin prick in the upper extremities bilaterally.
Given the raised concerns for cervical myelopathy, she was sent for an urgent MRI of the cervical spine. Mid-Sagittal T2 images (Figure 2A) revealed multilevel degenerative changes of the IVDs with significant compression of the cord at the C4/5, C5/6 and C6/7 levels with T2-hyperintense myelopathic signal changes. Given her MRI findings and her clinical presentation, she was diagnosed with a mJOA 12 cervical myelopathy. This patient was offered a 3-level ACDF which was done successfully. At 1-year follow-up, the patient was no longer reporting any pain, paresthesia or difficulties with hand motor function but was noted to have continued gait disturbance. Her post-operative mJOA was 16. A postoperative mid-sagittal T2 MRI scan shows a sufficiently decompressed spinal cord (Figure 2B), while the radiograph at follow-up shows an intact anterior plate and evidence of osseous fusion, (Figure 2C).

Figure 2: Illustrative case of a 62-year-old female patient with the diagnosis of a Degenerative Cervical Myelopathy. (A) The preoperative T2-sagittal magnetic resonance imaging shows a multi-level degenerative disc disease at the level of C4/5, C5/6 and C6/7 causing spinal cord compression and myelopathic signal alterations of the cervical spinal cord. Her preoperative mJOA score was 12. (B) The postoperative sagittal T2-MRI at follow-up shows a decompressed spinal cord and reduced myelopathic signals. (C) The postoperative radiograph at 1-year follow-up shows an intact ventral plate and signs of osseous fusion. On follow-up, the patient improved to an mJOA of 16.
Conclusion
The early diagnosis and management of DCM is paramount and requires a personalized multidisciplinary approach to care. DCM can have subtle and varied presentations with a range of underlying pathophysiology. Given the importance of early diagnosis, clinicians need to be attuned to early presenting signs of DCM and quick to complete workup. With advancements in imaging modalities, chemical biomarkers, and clinical ancillary testing in the near future, we can expect a complete personalized approach to the diagnosis of DCM that would enhance sensitivity of diagnosis and disease progression.
The treatment and clinical decision-making for patients with DCM depends on the severity of the disease and patient-specific factors. With the current evidence, there is clinical equipoise between operative and nonoperative intervention in mild DCM. As such, patient-specific factors and wishes would play a dominant role in the eventual treatment modality. The necessity of better understanding the uncertainties and needs of patients with a lived experience of DCM has been investigated in a recent online poll. Reference Davies, Mowforth and Khan107 This multi-stakeholder consensus process, termed AO Spine Research objectives and Common Data Elements for Degenerative Cervical Myelopathy (RECODE-DCM), aims to promote research efficiency in DCM through generation and ranking of research questions. An essential component to this process was found to be the inclusion of people with cervical myelopathy. Eventually, treatment outcomes can be enhanced in all patients by incorporating a personalized approach. The operative approach, pre- and post-operative care can be optimized to ultimately enhance patient outcomes.
Disclosures
The authors have nothing to disclose.
Acknowledgements
NH would like to acknowledge support from the Research Fund of the University of Basel for Excellent Junior Researchers. MGF acknowledges support from the Gerry and Tootsie Halbert Chair in Neural Repair and Regeneration.
Statement of Authorship
N.H.: conception and design, collection and/or assembly of data, data analysis and interpretation, manuscript writing, final approval of manuscript; A.M.: collection and/or assembly of data, data analysis and interpretation, manuscript writing, final approval of manuscript; N.M.: manuscript writing, final approval of manuscript; M.G.F.: conception and design, manuscript editing, final approval of manuscript.