Human milk is the optimal food for infants, but breast-feeding is not always possible or desired. In European countries, between 56 % and 98 % of infants are breastfed directly after birth, but only 38 % to 71 % are breastfed up to 6 months(Reference Theurich, Davanzo and Busck-Rasmussen1). Hence, a large proportion of infants receive infant formula during their first months of life. Infant formulas are the most adequate breastmilk substitute because of their suitability to cover the infant’s nutritional requirements(Reference Martin, Ling and Blackburn2). To match the casein to whey protein ratio of human milk (40:60 on average), most infant formulas are formulated using skim bovine milk supplemented with bovine whey proteins. Lactose, lipids generally of vegetable origin, minerals and vitamins are also added to mimic as closely as possible the nutritional composition of human milk(Reference Floris, Lambers, Alting and Griffiths3). The manufacturing process of infant formula powder includes a succession of thermal processes such as pasteurisation, evaporation and spray drying to ensure microbiological safety and shelf life stability. However, these successive thermal treatments cause several modifications of the native proteins, such as denaturation and aggregation, and promote the generation of Maillard reaction products(Reference Brodkorb, Croguennec, Bouhallab, McSweeney and O’Mahony4,Reference Yu, Leconte and Mejean5) .
The physicochemical changes occurring during the industrial processes of infant formulas can in turn affect milk protein digestibility, which may lead to a reduction in their nutritional quality(Reference van Lieshout, Lambers and Bragt6). Notably, heat treatments have been reported to affect the in vitro digestion kinetics and the digesta microstructure, especially during the gastric phase. Studies have observed increased or slowed down protein hydrolysis depending on the protein considered (caseins or ß-lactoglobulin) and heat-induced protein structure modifications(Reference Dupont, Boutrou and Menard7–Reference Sánchez-Rivera, Ménard and Recio9). For instance, it has recently been shown, using an in vitro static infant digestion model, that heat treatment led to enhanced gastric hydrolysis of caseins due to modification of the micelle structure(Reference Halabi, Croguennec and Bouhallab10). In vivo, thermal treatments of milk proteins also induced differences in protein digestion kinetics, mainly due to different gastric behaviours(Reference van Lieshout, Lambers and Bragt6). For example, in pigs, heating milk increased its mean retention time in the stomach and decreased ß-lactoglobulin resistance to gastric hydrolysis(Reference Barbe, Menard and Le Gouar11). In humans, accelerated milk digestion kinetics were observed with ultra-high temperature milk compared with unheated milk, leading to lower dietary nitrogen retention(Reference Lacroix, Bon and Bos12). These differences in terms of digestion kinetics may not always be associated with modifications in overall digestibility(Reference Efigênia, Povoa and Moraes-Santos13,Reference Lacroix, Leonil and Bos14) . Nevertheless, some studies have shown that heat treatment decreased protein or amino acid digestibility compared with unheated milk products in rats(Reference Lacroix, Leonil and Bos14–Reference Rutherfurd and Moughan16). Altogether, these results suggest that heat treatments influence the digestion kinetics of milk proteins and thus may modify the nutritional quality of milk-based products. In addition, as the protein content of infant milk formulas is lower and the casein amount is half of that in bovine milk, infant formulas may behave differently from standard milk-based products to heat treatments.
Recently, minimally processed infant formula has been developed(Reference Yu, Leconte and Mejean5). The innovative processing route was based on membrane filtration of fresh milk for debacterisation and whey protein purification coupled to low-temperature unit operations (evaporation and spray drying) that allowed the production of minimally processed and bacteriologically safe infant formula powder. This infant formula contained virtually only native proteins (94 %) unlike that in classically produced infant formula (58 %), as well as a tendency towards slightly fewer Maillard reaction products(Reference Yu, Leconte and Mejean5). In an in vitro digestion study, an infant formula produced by cascade membrane filtration has been shown to present a lower degree of proteolysis at the end of the gastric phase compared to heat-treated infant formula, with a similar degree of protein hydrolysis at the end of the intestinal digestion(Reference Bavaro, Mamone and Picariello17). Similar trends were observed for the present minimally processed infant formula, which presented larger aggregated particles in the gastric phase and reduced proteolysis for whey proteins as compared with heat-treated infant formulas (Deglaire et al. unpublished results). When investigating an unheated but defatted infant formula in an in vitro dynamic digestion model, the overall protein digestibility was reduced at the end of the gastro-intestinal digestion(Reference Halabi, Croguennec and Ménard18). Minimally processed and heat-treated infant formulas may thus display different protein digestion kinetics, which may affect protein digestibility and quality. However, so far, little information is available regarding the protein digestion kinetics of minimally heat-treated infant formula in vivo.
In this context, our study aimed to evaluate the protein digestion kinetics and protein quality of a minimally processed infant formula (T−) compared with an infant formula receiving several and higher heat treatments (T+++), similarly to commercially available infant formulas. For this purpose, the protein and amino acid true digestibility, the postprandial nitrogen intestinal kinetics and the postprandial plasma amino acid kinetics were evaluated in young rats after ingestion of diets containing either T− or T+++ infant formulas.
Materials and methods
Animals
This animal study was conducted in compliance with the EU directive 2010/63/EU for animal experiments and ARRIVE guidelines. It was approved by the Ethics Committee in Animal Experiment of INRAE (n° 20–06, Jouy-en-Josas, France) and the French Ministry of Higher Education and Research (APAFIS#25 035–20200421180188 v1). Sixty-eight male Wistar Han rats aged 21 d (Envigo, Gannat, France) were included in the study.
Diets
Two infant formula powders were tested in the study: T− formula, which was produced with minimal processing routes, and T+++ formula, which was produced with successive heat treatments. They were produced at a semi-industrial scale at UMR STLO (Rennes, France) based on ingredients obtained by fresh bovine milk microfiltration as described in Yu et al. 2021(Reference Yu, Leconte and Mejean5). Briefly, T− formula was produced through low-temperature vacuum evaporation (50°C) and spray-drying (inlet and outlet temperatures of 160 and 70°C, respectively), with no additional heat treatments, whereas successive heat treatments (72°C for 30 s; 90°C for 2–3 s before evaporation; 85°C for 2 min before spray-drying) were additionally conducted to produce T+++ formula, mimicking a commercial powdered infant formula. The compositions of the infant formulas are detailed in Yu et al. 2021(Reference Yu, Leconte and Mejean5). The T+++ and T− infant formulas were only included up to 40 % in the diets, and other ingredients were added (total milk protein, sucrose, starch, soya oil cellulose, vitamins and minerals) in order to obtain AIN-93G-modified diets(Reference Reeves, Nielsen and Fahey19) that match young rat nutritional requirements (Table 1). The control diet was an AIN-modified standard milk protein diet for young rats, but with adjustments to the lactose and lipid quantities in order to match the lactose and lipids provided by the infant formula in the T+++ and T− diets. The diets were isoenergetic (17·2 kJ/g) and contained 20 % of energy from proteins, 53 % from carbohydrates and 26 % from lipids.
Table 1. Composition of the experimental diet (in g/kg)
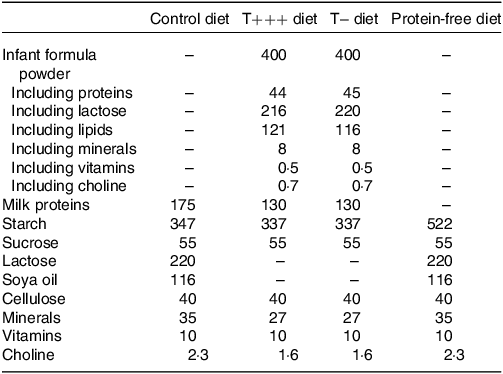
Added minerals and vitamins contribute to 45 mg per kg of final diet.
Experimental procedure
Sixty-eight rats were included in the study and housed under controlled conditions (12-h light/dark cycle, lights off 08.00–20.00, room temperature of 22°C) in individual cages with wire bottoms to prevent coprophagia. They were adapted over 1 week to the animal facility conditions and fed the control diet during this week. Then, they were randomly split into three groups corresponding to their diet (Table 1, online Supplementary Table 1): control (n 8), T+++ (n 30) and T− (n 30). The rats were fed the control, T+++ or T− diets for 2 weeks (Fig. 1), and energy intake and body weight were recorded every day (except during the week-end). During the first week, the experimental diets were available ad libitum 24 h a day. However, during the second week, the experimental diets were available ad libitum from 11.00 to 17.00, and the rats were trained to eat a small 3-g meal of the diet at 08.00 for 30 min. At the end of the 2 weeks, the control rats were given a 3-g protein-free meal and euthanised 6 h after meal ingestion to evaluate the endogenous nitrogen and amino acid intestinal losses. The T− and T+++ rats received a 3-g meal corresponding to their diet and were sequentially euthanised at 0 (n 6), 1 (n 6), 2 (n 6), 3 (n 6) and 6 h (n 6) after meal ingestion to obtain kinetics of plasma parameters and N content in the digestive segments. Rats have no access to other food than the test meal before euthanasia. Rats were euthanised by intracardiac puncture under isoflurane anaesthesia. Five mL of blood was collected from the intracardiac puncture into tubes with heparin, kept on ice for 10 min and centrifuged (3000 g, 15 min, 4°C) for plasma collection. Plasma was stored at −80°C for subsequent analyses. Gastrointestinal segments were identified as the stomach, the small intestine (stomach to ileocaecal junction), the caecum and the colon (from the end of the caecal ampulla part to the anus). The luminal contents of the intestinal segments were rinsed with NaCl solution (9‰), collected entirely and stored at −20°C before being dry-frozen. Finally, parameters of body composition were determined. The liver, the spleen and the kidneys were removed and weighed. Abdominal fat pads (mesenteric, retroperitoneal, epididymal and subcutaneous) were excised and weighed to evaluate fat mass, and the carcass was stripped to assess fat-free mass.
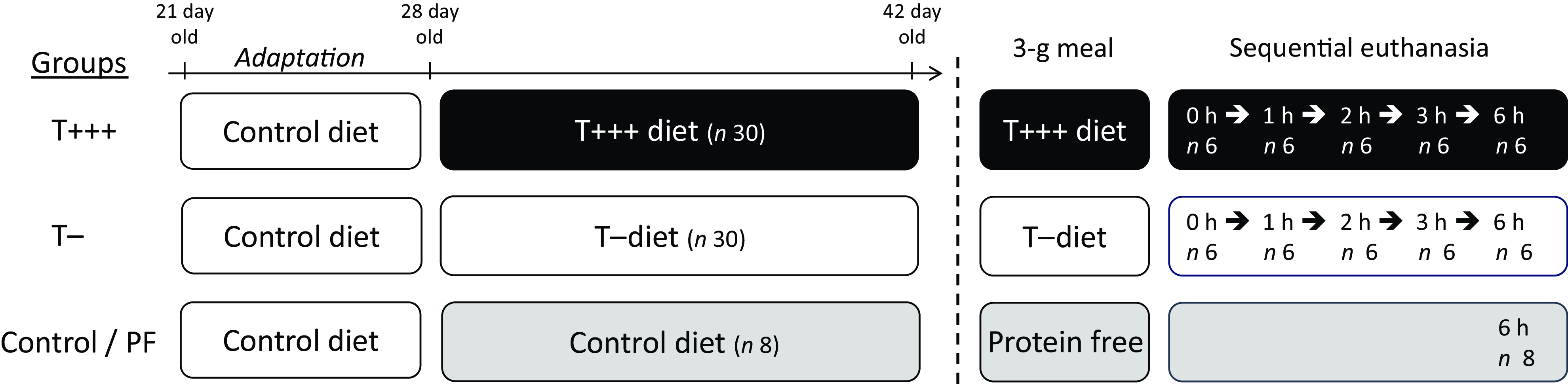
Fig. 1. Schematic representation of the experimental protocol.
Analytical procedures
Total nitrogen in digestive contents or diets was determined by the Dumas method using an elemental analyser (Vario Micro Cube; Elementar), with atropine as the elemental standard.
For amino acid quantification (except tryptophan), 5–10 mg of digestive contents or diets were hydrolysed with HCl 6N for 24 h at 110°C, and norvaline was used as an internal standard, added before hydrolysis. For the analysis of sulphur amino acids, performic acid oxidation was carried out before hydrolysis in order to convert methionine and cysteine to the acid-stable derivatives (methionine sulfone and cysteic acid, respectively)(Reference Rutherfurd and Gilani20). For tryptophan analysis, 15 mg of digestive contents were hydrolysed for 20 h with barium hydroxide 2N at 110°C, and 5-methyl-tryptophan was used as an internal standard, added before hydrolysis. Calibration standards were composed of an amino acid mixture (Waters), to which specific amino acids were added (norvaline, methionine sulfone, cysteic acid, tryptophan and 5-methyl-tryptophan). Hydrolysates and standards were then derivatised using the AccQTag Ultra Derivatization Kit (Waters) according to the manufacturer’s protocol. The amino acid analysis was performed on an Acquity HClass ultra-high performance liquid chromatography system with a photodiode array detector (PDA detector; Waters). The amino acids were separated using an AccQ-Tag AA C18 column (2·1 × 100 mm; 1·7 μm bead size; Waters) and quantified as mmol/g of dry matter. External standards (BSA for all amino acids except tryptophan and lysozyme for tryptophan) are used in each sample batch to verify the recovery of amino acids during hydrolysis.
Amino acid plasma concentrations were determined by ultra-high performance liquid chromatography on samples deproteinised by the addition of sulfosalicylic acid, with norvaline as an internal standard, and after derivatisation with the AccQTag Ultra Derivatization kit (Waters). Blood glucose was immediately assayed using a standard glucometer (Onetouch Vita, Lifescan). Urea content in plasma was assessed with a urea assay kit (Urea assay, Randox) based on the Urease-Berthelot method. Plasma insulin and TAG were measured using commercial kits (Rat Insulin ELISA, Mercodia, Uppsala, Sweden, and TAG Assay, Randox, Roissy-en-France, France, respectively).
Calculation
True orocaecal nitrogen and amino acid digestibilities were determined in rats euthanised 6 h after meal intake. The collection of digesta 6 h after meal ingestion facilitates a compromise between complete digestion and a minimal duration of caecal fermentation(Reference Guillin, Gaudichon and Guerin-Deremaux21). Orocaecal nitrogen digestibility was calculated as follows:

where N ingested is the quantity of nitrogen ingested from the meal (in mmol), Ncaecum total is the total nitrogen content (in mmol) in the caecum of T+++ and T− rats, and Ncaecum endogenous is the endogenous loss of nitrogen in the caecum (in mmol). Endogenous losses were determined in the control group following ingestion of a protein-free meal. True orocaecal digestibility was calculated for each individual amino acid with the same formula as nitrogen.
The composition in amino acids of the T+++ and T− infant formulas is presented in online Supplementary Table 1. The chemical score of T+++ and T− infant formulas was determined as previously described(22). The digestible indispensable amino acid score (DIAAS) of T+++ and T− infant formula was determined as the lowest digestible indispensable amino acid ratio (DIAAR)(22) and DIAAR were calculated for each amino acid as follows:

where digestible IAAi content corresponds to the indispensable amino acid i content of the infant formula T+++ or T− corrected by the true orocaecal digestibility of this amino acid. The reference profile used for the chemical score and DIAAS calculation was the requirement pattern for the 0-6 m children(22).
Statistical analysis
A power calculation was performed to determine the sample size required to detect significant differences in protein digestibility between two groups with a statistical power of 90 % and α level set at 0·05. According to former comparable studies, inter-individual variability in protein digestibility measured at ileal or caecal level in rats was around 1·2 %, and the difference in digestibility between 2 groups was about 2·5 %(Reference Guillin, Gaudichon and Guerin-Deremaux23,Reference Rutherfurd, Fanning and Miller24) , leading to a sample size of n 6/group (G * Power 3·1).
Values are presented as means ± sd. Statistical analyses were performed using GraphPad Prism Version 9·3·1 (GraphPad Software). According to Quantile vs. Quantile Plots and Shapiro Wilk tests(Reference Ghasemi and Zahediasl25), the data were assumed to be normally distributed. Body weight and energy intake were analysed with a mixed model with group as a fixed factor and time as the repeated factor(Reference Gałecki, Burzykowski, Casella, Finenberg and Olkin26). Plasma kinetics and N content in the different segments were analysed with a two-way ANOVA to detect the main and interactive effects of the group and time after meal intake. Comparisons between groups were analysed using a one-way ANOVA or an unpaired t test(Reference Mishra, Singh and Pandey27). Post hoc Bonferroni tests were applied for pairwise comparisons. Differences were considered statistically significant at P < 0·05.
Results
Energy intake, body weight and body composition
Significant effects of time (P < 0·001) and time and group interaction (P < 0·001) were observed for energy intake among the three groups (control, T− and T+++ diets) during the 2 weeks of nutritional intervention (Fig. 2(a)). However, no difference between groups at each time point was revealed by post hoc comparisons. The change in the dietary intake pattern (from ad libitum 24 h/d to a small meal at the beginning of the dark phase and 6 h of ad libitum food access) on day 8 induced a decrease in energy intake (day 8 vs. day 1–5, P < 0·001); but as soon as day 10, energy intake returned to the normal level (day 10 vs. day 1–4, P > 0·05). A significant effect of time was revealed for the body weight (P < 0·001) with no effect of group and a tendency towards an interaction effect of time and group (P = 0·06) (Fig. 2(b)). As for energy intake, a reduction in body weight gain was observed on day 8 due to the change in dietary intake pattern. Despite no group effect on the body weight evolution, 2 weeks of diet including the infant formula induced a significantly higher body weight gain (Table 2) in T− and T+++ rats compared with control rats (P = 0·02, for both). Furthermore, the analysis of body composition at euthanasia revealed a significantly higher fat-free mass for the rats consuming the T− or T+++ diets compared with control rats (P = 0·002 and 0·007, respectively), while no difference was observed for fat mass, naso-anal length, femoral bone mineral density or the weight of several organs. The weight of the kidneys was higher in the T− group in comparison to the control group (P = 0·04). No difference between groups was observed for blood glucose kinetics, plasma insulin, urea or TAG after meal intake (online Supplementary Fig. 1).
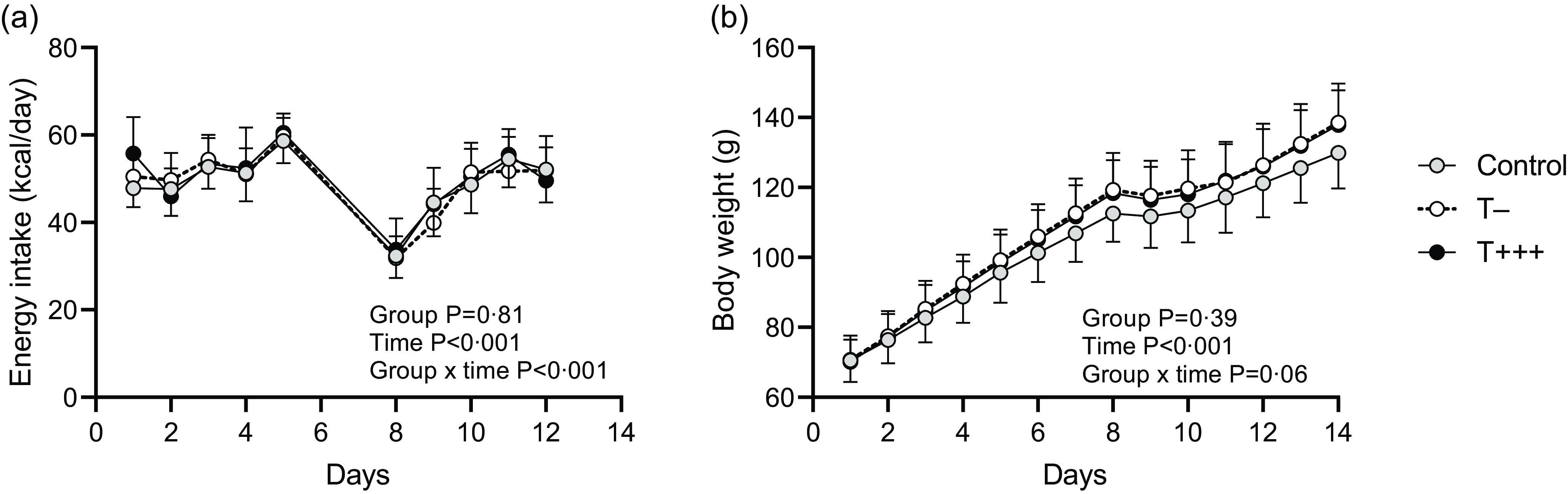
Fig. 2. Daily energy intake (a) and body weight (b) during the 2-week nutritional intervention. From day 1 to day 7, the rats received food ad libitum during 24 h per day and from day 8 to day 14, they received a small meal (3 g) during 30 min in the morning and food ad libitum from 11.00 to 17.00. Values are means ± sd, with n 30/group for T+++ and T− groups and n 8 for control group.
Table 2. Body and tissue composition after 2 weeks of infant formula diets
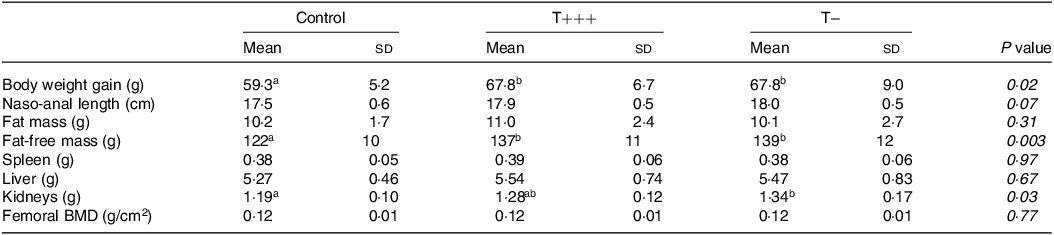
n 30 for T+++ and T− groups and n 8 for control group. Differences between groups were tested using a 1-factor ANOVA, and different letters indicate significant differences between groups (Bonferroni post hoc tests). Orthogonal contrasts between T+++ and T− groups were also performed to specifically compare these two groups, but no difference was observed.
BMD, bone mineral density.
Protein digestibility
The total nitrogen content in the different parts of the intestinal tract after meal ingestion was determined in all rats. Total nitrogen kinetics were determined owing to sequential euthanasia of T− and T+++ groups and are presented in Fig. 3. Due to stomach emptying, a significant effect of time on nitrogen content in the stomach was found (P < 0·001), with a gradual decrease of nitrogen content from 1 h to 6 h after meal intake. However, no difference between T− and T+++ rats was observed. The nitrogen content in the small intestine or caecum was stable over time, and no difference between T− and T+++ groups was observed. In the colon, a decrease in nitrogen content as a function of time following meal intake was observed (P < 0·001), but it was comparable between groups.
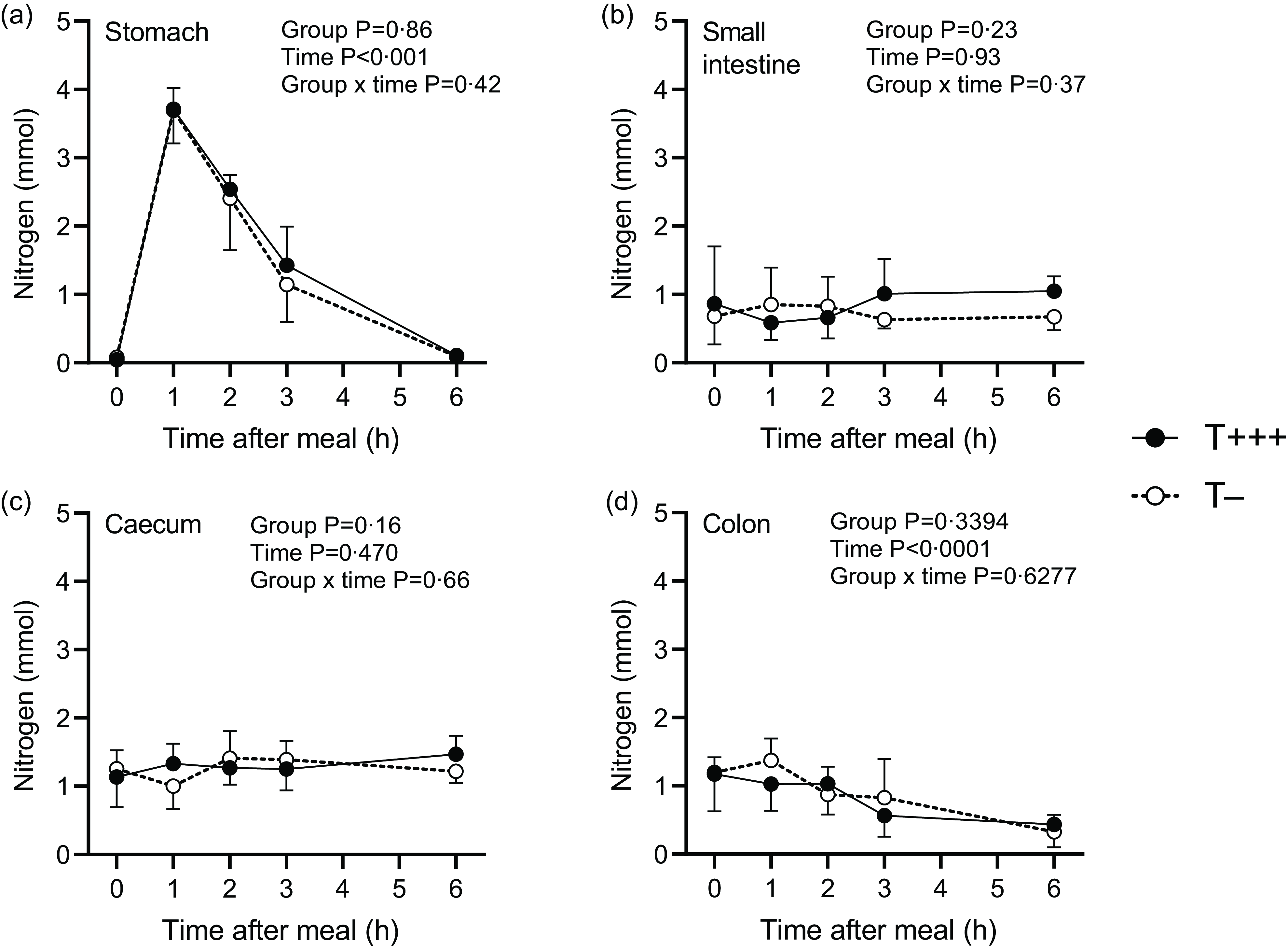
Fig. 3. Kinetic patterns of total nitrogen content in the stomach (a), the small intestine (b), the caecum (c) and the colon (d) after ingestion of T+++ and T− meals. Values are means ± sd, with n 6/group for each time point.
The rats in the control group ingested a protein-free meal and were euthanised 6 h later to quantify endogenous nitrogen in the upper part of the digestive tract. Rats from this group were euthanised 21 h after their last protein intake (15 h of fasting + 6 h after ingestion of a protein-free meal). It was thus estimated that the caecum did not contain any significant amount of dietary proteins, but colon digesta might still contain dietary proteins(Reference Varga28), therefore endogenous losses were not estimated in the colon. Endogenous nitrogen losses were 0·16 ± 0·07 mmol, 1·13 ± 0·25 mmol and 1·06 ± 0·23 mmol in the stomach, small intestine and caecum, respectively. Dietary nitrogen in the same gastrointestinal compartments of the T+++ and T− rats euthanised 6 h after meal intake was then determined (Table 3). The majority of the dietary nitrogen was found in the caecum. No difference between groups was observed for dietary nitrogen recovery in the stomach and small intestine 6 h after meal ingestion, but there was a tendency towards more dietary nitrogen in the caecum of rats consuming the T+++ meal compared to the rats consuming the T− meal (P = 0·09). Consequently, orocaecal nitrogen digestibility of the T− diet tended to be higher than for the T+++ diet (96·6 ± 3·1 % and 91·9 ± 5·4 %, for T− and T+++, respectively; P = 0·09).
Table 3. Dietary nitrogen recovered in gastrointestinal contents and nitrogen digestibility in young rats 6 h after ingestion of T+++ and T− diets
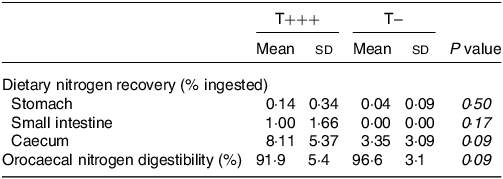
n 6 /group. Differences between groups were tested using an unpaired t test. Dietary nitrogen was determined in the different compartments by subtracting endogenous nitrogen (estimated in the control group after consumption of a protein-free meal) to total nitrogen content.
Amino acid digestibility
Amino acid digestibility of the T− and T+++ diets was determined using dietary amino acid recovery in the caecum of rats euthanised 6 h after meal ingestion (Table 4). To calculate the true orocaecal digestibility, endogenous amino acid losses were calculated using the control group consuming a protein-free meal (online Supplementary Table 2). The mean true orocaecal digestibility of amino acids was 91·4 ± 5·2 % for T+++ and values ranged from 85·5 ± 7·8 % for glycine to 95·9 ± 4·5 % for arginine. For T−, the mean true orocaecal digestibility of amino acids was 94·9 ± 3·6 % and values ranged from 90·0 ± 5·7 % for serine to 98·6 ± 1·7 % for arginine. For all amino acids, the orocaecal digestibility of T+++ and T− diets was not different.
Table 4. Orocaecal amino acid digestibility of T+++ and T− diets determined in young rats 6 h after meal ingestion
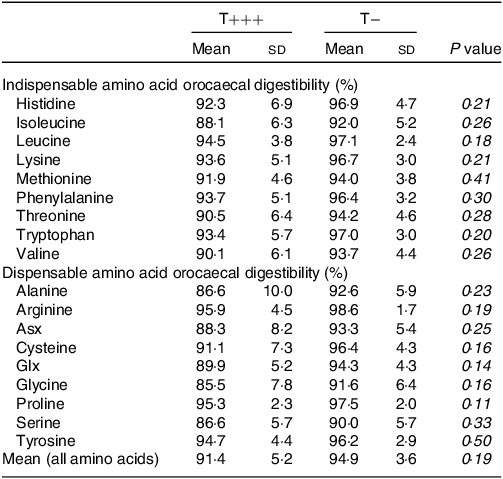
Asx, aspartate + asparagine; Glx, glutamate + glutamine; n.s., non-significant.
n 6/group. Mean amino acid digestibility was calculated from amino acid digestibilities weighted by the proportion of each amino acid in the T+++ or T− diet. Differences between groups were tested using an unpaired t test.
Based on their amino acid compositions (online Supplementary Table 1), the chemical score of the T− and T+++ infant formulas was calculated (Table 5). Chemical scores of 0·87 for T+++ and 0·83 for T− were obtained due to low aromatic amino acid content. The true orocaecal amino acid digestibility of the T+++ and T− diets allowed the calculation of the DIAAS of the T+++ and T− infant formulas (Table 5). The DIAAS was 0·82 for T+++ and 0·80 for T−, with the limiting amino acids being aromatic amino acids for both infant formulas. Isoleucine was the secondary limiting amino acid.
Table 5. Chemical score and DIAAS of T+++ and T− infant formula
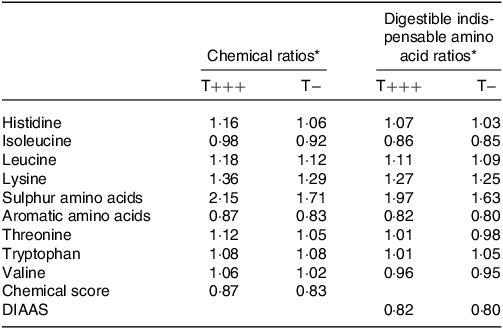
DIAAS, digestible indispensable amino acid score.
* The chemical scores and DIAAR were calculated using the amino acid requirement pattern for the 0–6 m children according to the FAO 2013(22).
Plasma amino acid concentrations
Plasma amino acid concentrations were determined in all rats. The kinetics of plasma indispensable and total amino acids are presented in Fig. 4. A significant effect of time (P < 0·001) and group (P < 0·001) influenced total and indispensable plasma amino acid kinetic patterns. An initial increase was observed 1 h after meal intake for T− diet (P = 0·003 and P < 0·001, 1 h vs. 0 h for indispensable and total amino acids, respectively) and 2 h after meal intake for the T+++ diet (P = 0·03, 2 h vs. 0 h for total amino acids), followed by a return to basal level 3 h after meal intake for both diets. The initial increase in plasma amino acid concentration was significantly higher after T− diet intake than T+++ (P = 0·01 for indispensable amino acids and P < 0·001 for total amino acids). Similar patterns were observed for dispensable amino acids and individual amino acids such as alanine, asparagine, phenylalanine, proline, serine, threonine, tryptophan and tyrosine (data not shown).
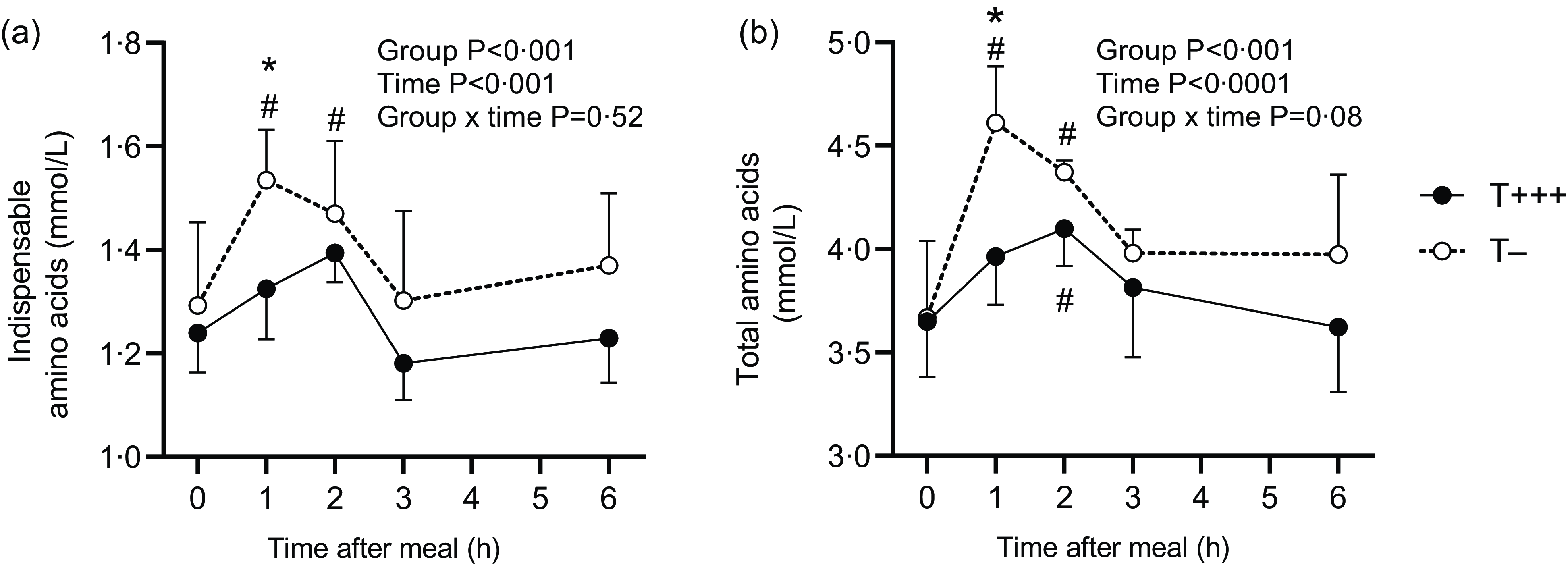
Fig. 4. Plasma concentrations of indispensable amino acids (a) and total amino acids (b) in rats after ingestion of T+++ and T− meals. Values are means ± sd, with n 6/group for each time point. *Indicates significant differences between T+++ and T− groups for a specific time point. # indicates significant differences with basal level (0 h after meal) within each group.
Discussion
This study compared the in vivo nitrogen and amino acid bioavailability of a minimally processed infant formula, presenting a low protein denaturation extent, to a standard infant formula receiving successive heat treatments. Infant formula powders could be incorporated only up to 40 % into young rat’s (1-month-old) diet. Hence, the experimental diets contained not only proteins, carbohydrates and lipids from the infant formula powders but also other ingredients (total milk proteins, sucrose, starch, soy oil, cellulose, etc.) in order to fulfill rat requirements. Despite the limited contribution of the infant formula to the rat diet, the minimally processed infant formula tended to increase nitrogen digestibility, and a significantly more rapid and higher plasma amino acid level was reported after meal ingestion.
Young rats were first fed for 2 weeks with either the T− diet, the T+++ diet, or the control diet. The diets were formulated to meet the nutritional requirements of rats during growth. Daily energy intake and body weight evolution were similar between groups, suggesting that heat treatments applied to infant formula did not alter its capacity to support rat growth, as previously observed(Reference Efigênia, Povoa and Moraes-Santos13). Control rats displayed, however, a slightly lower final body weight due to lower fat-free mass. The casein to whey protein ratio of rat milk is 40:60, as for human milk. The experimental T− and T+++ diets had a casein to whey protein ratio of 70:30 since they were composed of infant formulas and total milk proteins. It is possible that these diets were more adapted in terms of protein composition for 1-month-old rats than the control diet containing only total milk proteins (80:20).
After 2 weeks, the rats were euthanised after ingestion of a small meal containing either the T− or the T+++ diet. In the stomach, gastric emptying of total nitrogen was similar for both diets, with a gradual decrease from 1 h to 6 h after meal intake, unlike differences reported for pigs or humans ingesting raw or heat-treated milk(Reference Barbe, Menard and Le Gouar11,Reference Lacroix, Bon and Bos12) . This might be due to the limited contribution of T+++ and T− to the diet (40 %) in the present study, which may have mitigated the possible differences. No difference was observed in nitrogen content kinetics in the small intestine, caecum, or colon. During the post-prandial phase, the dynamic process of dietary and endogenous nitrogen entering the intestinal lumen and being absorbed by the mucosa may explain the stability over time of total nitrogen content in the small intestine, as previously observed(Reference Buraczewski, Porter and Rolls29). The caecum accumulates the digesta leaving the small intestine before it enters the colon. While it has been previously shown that dietary nitrogen increased in the caecum after meal intake(Reference Oberli, Douard and Beaumont30), the total nitrogen content did not vary since the quantity of dietary nitrogen was small for proteins of high digestibility. Only very small amounts of dietary nitrogen entered the colon in the 6 h following meal intake(Reference Calvez, Khodorova and Beaubier31,Reference Atallah, Gaudichon and Boulier32) while feces from the previous meals came out, explaining the decrease in nitrogen content with time.
Six hours after T− and T+++ meal ingestion, dietary nitrogen and amino acid recovery were determined along the upper gastrointestinal tract. Protein and amino acid endogenous losses were estimated in the control group euthanised 6 h after ingestion of a protein-free meal. Orocaecal digestibility was determined as a proxy for ileal digestibility, as previously done(Reference Lacroix, Leonil and Bos14,Reference Guillin, Gaudichon and Guerin-Deremaux23,Reference Oberli, Douard and Beaumont30,Reference Tessier, Khodorova and Calvez33) . This method consists of collecting quantitatively the cecal digesta during a limited post-digestion period (usually 6 h), allowing a compromise between complete digestion of the test meal and minimal duration of fermentation. Comparable digestibility values have been obtained at the ileal and caecal levels in rats for different protein sources(Reference Guillin, Gaudichon and Guerin-Deremaux21). In the present study, high nitrogen and amino acid true orocaecal digestibilities were found for both T− and T+++ diets, with values above 90 %. Since T− and T+++ diets have similar total milk protein content, the digestibility differences found between T− and T+++ diets rely on the quality of infant formula proteins. These values are consistent with those reported in the literature for milk proteins in rat studies(Reference Lacroix, Leonil and Bos14,Reference Rutherfurd, Fanning and Miller24,Reference Atallah, Gaudichon and Boulier32) . Slightly higher nitrogen and amino acid true ileal digestibility values (98 %) of the heat-treated infant formula T+++ have been reported in piglets(Reference Charton, Henry and Cahu34), which might be due to the animal model. Despite the fact that no significant difference was reported, orocaecal nitrogen and amino acid true digestibility of the T+++ diet tended to be lower than for the T− diet. The absence of any significant difference may result from the relatively high inter-individual variability but also from the limited contribution of the infant formulas to the rat diet (40 %), as previously mentioned. Digestibility loss following heat treatments of dairy products has previously been observed. In rats, spray-dried milk displayed a slightly lower true orocaecal nitrogen digestibility than microfiltered milk, as performed for the present T− infant formula(Reference Lacroix, Leonil and Bos14). Heat treatments (121°C, 10 min, or 1 h) applied to skim milk have previously been shown to decrease true orofaecal nitrogen digestibility as well as true ileal amino acid digestibility in young rats(Reference Gilani and Sepehr15,Reference Rutherfurd and Moughan35) . The heat-reduced digestibility of dairy products may be related to the formation of Maillard reaction products that block amino acid residues and the formation of aggregates between caseins and denatured whey proteins that can reduce the access of digestive enzymes(Reference Halabi, Croguennec and Bouhallab10,Reference Cattaneo, Stuknyte and Masotti36,Reference Pinto, Léonil and Henry37) . The T− infant formula produced with minimal heat treatments presented a protein denaturation extent significantly lower than for T+++ and a slightly lower content of Maillard reaction products (8 and 10 mg of Nε-carboxymethyl lysine/100 g of proteins in T− and T+++, respectively)(Reference Yu, Leconte and Mejean5). Hence, the thermal treatments applied to the T+++ infant formula modified the protein structure, which in turn seemed to affect digestibility. The digestibility of lysine was numerically lower for T+++ than for T− but this did not reach statistical significance. A similar result was observed when comparing highly heat-treated infant formula and native human milk(Reference Charton, Henry and Cahu34). The determination of reactive lysine could have provided more information regarding lysine digestibility values. In a rat study evaluating digestible reactive lysine in milk products, higher digestibility was found for reactive lysine compared with total lysine in infant formulas(Reference Rutherfurd and Moughan38).
Regarding the protein quality of the minimally processed and the heated infant formulas, comparable DIAAS values around 0·8 were obtained for T− and T+++ due to low aromatic amino acid content, especially tyrosine. Analyses of the amino acid composition of infant formulas have previously reported limited contents of aromatic amino acids, isoleucine, histidine and/or tryptophan(Reference Maathuis, Havenaar and He39–Reference Almeida, Baião and Leandro41). Moreover, the values obtained for the DIAAS were in line with previously reported ones. T+++ infant formula displayed a DIAAS of 0·83 (for aromatic amino acids) when assessed in piglets(Reference Charton, Henry and Cahu34) and an in vitro dynamic model simulating infant digestion reported DIAAS values of 0·75 (for aromatic amino acids) for an infant formula(Reference Maathuis, Havenaar and He39). A small decrease in lysine content was observed in infant formulas when considering total or reactive lysine (0–0·3 g/100 g of protein)(Reference Rutherfurd and Moughan38). Since the DIAAR of lysine of T− and T+++ were > 1·2, it is unlikely that the DIAAS values of T− and T+++ would have been different when considering reactive instead of total lysine. The present study, in accordance with previous studies, showed that the DIAAS values were related to the low aromatic amino acid content, while the high digestibility had a moderate influence on these protein quality scores.
Plasma amino acid kinetics were also evaluated after ingestion of the T− and T+++ diets. Plasma indispensable and total amino acids were found to increase postprandially after both diets, but this increase was higher and more rapid after ingestion of the T− meal. Such results have previously been observed in piglets fed native or denatured whey protein solutions(Reference Welch-Jernigan, Abrahamse and Stoll42). In contrast, in preterm infants, intake of fresh human milk induced a slower and lower increase in plasma indispensable amino acid concentration than intake of infant formula(Reference Moro, Minoli and Boehm43). However, various factors may explain such a difference, including not only the different heat treatment level but also the different food structure and the different protein profile with different proteolysis susceptibility. The earlier and higher increase in plasma amino acids after intake of the minimally processed infant formula observed in the present study may be related, at least partly, to its 5 % higher digestibility, resulting in plasma amino acid content being 10–15 % higher at the maximum. It may also suggest that the two diets possess different digestive kinetics, although this was not revealed by the quantification of total nitrogen in the stomach and the whole small intestine. Since the modulation of amino acid kinetics markedly influences the metabolic fate of dietary nitrogen(Reference Lacroix, Bos and Leonil44), further studies are needed to explore the postprandial utilisation of dietary amino acids following ingestion of minimally processed or heat-treated infant formulas.
In conclusion, this pre-clinical study showed that minimal processing routes to produce minimally processed infant formula did not modify protein quality determined with the DIAAS and even tended to enhance its true nitrogen digestibility and increase postprandial plasma amino acid concentration. Further investigations are needed to confirm that preserving the native structure of proteins within infant formula would modulate postprandial protein metabolism. Finally, in addition to providing high quality proteins with less affected structure, the minimally heated processing may be exploited in order to preserve heat-sensitive proteins in infant formula, notably the ones that present interesting biological activities for the infant.
Acknowledgements
We thank Morgane Dufay from UMR PNCA for her contributions to animal experiments, as well as our intern Héloïse Eliet for her contributions to animal experiments and analyses. We thank Xiaoxi Yu, Nadine Leconte, Serge Méjean and Gilles Garric from STLO for the production of infant formulas.
This research project was funded by the Institute Carnot Qualiment.
The authors’ responsibilities were as follows: J. C., C. G.: designed the research; A. D.: supplied the T− and T+++ infant formulas; J. C., A. B. and A. M. D.: conducted the research; J. C.: analysed the data and wrote the first draft of the paper and all authors: reviewed and edited the manuscript and approved the final manuscript.
The authors declare that they have no known competing financial interests or personal relationships that could have appeared to influence the work reported in this manuscript.
Data described in the manuscript will be made available upon reasonable request, pending application and approval.
Supplementary material
For supplementary materials referred to in this article, please visit https://doi.org/10.1017/S0007114523002696