Nutrition and diet as environmental factors and determinants of growth and body composition can contribute to the risk of human cancers(Reference Granados, Quiles and Gil1–Reference Taghavi and Yazdi3). Evidence pertaining to the role of dietary factors in carcinogenesis comes from both epidemiological studies and laboratory experiments. Dietary fat has been known to cause cancer of different organs(Reference Woutersen, Appel and van Garderen-Hoetmer4). Vegetable oils are a major source of fat intake, and have been used as a common medium for cooking since ages. The practice of repeated heating of edible oils is associated with adverse health effects(Reference Ima-Nirwana, Ahmad and Yee5). High-temperature cooking techniques such as grilling and pan frying are associated with increased risk of carcinogenicity(Reference Sinha, Chow and Kulldorff6) and genotoxicity(Reference Li, Wang and Zhang7, Reference Dung, Wu and Yen8). Repeatedly heated fat possesses mutagenic potential(Reference Hageman, Kikken and Ten Hoor9), and increase in mutagenicity is proportionally related to its frying time(Reference Chen, Chiu and Chen10). A number of genotoxic responses caused by the dietary use of heated cooking oils have also been reported(Reference Li, Wang and Zhang7, Reference Chiang, Pei-Fen and Ying11). Studies from different sources have indicated that the heating of the vegetable oils at high temperatures leads to the formation of compounds having the potential to cause cancers(Reference Dung, Wu and Yen8, Reference Wu, Chiang and Wang12, Reference Wu and Yen13). The heat processing of the oils at high temperatures generates food contaminants such as polycyclic aromatic hydrocarbons (PAH), a family of toxic and mild to potent carcinogenic chemicals(Reference Ramesh, Walker and Hood14, Reference Pandey, Dhawan and Das15). The epidemiological study done by Lopez-Abente et al. (Reference Lopez-Abente, Sanz-Anquela and Gonzalez16) suggested that individuals exposed to PAH are at the risk of developing cancers. Diet is the major non-occupational source of PAH for non-smokers(Reference Lodovici, Dolara and Casalini17), with meat, meat products, cereals, oils and fats being the principal sources(Reference Ibáñez, Agudo and Berenguer18).
Epidemiological studies have indicated that a high intake of saturated fat increases the risk of cancers(Reference Caygill, Charlett and Hill19). Coconut oil (CO), also known as coconut butter, is a tropical oil extracted from copra (the dried inner flesh of coconuts) of Cocos nucifera (Family: Arecaceae), which is used as a cooking medium mainly in the southern parts of India and other South-East Asian countries. The CO contains predominantly medium-chain TAG, with 86·5 % SFA, 5·8 % MUFA and 1·8 % PUFA. The SFA of CO could have an effect on atherosclerosis not only beyond their role in affecting plasma lipoproteins, but also through increased production of inflammatory cytokines in the arterial walls(Reference Alexaki, Wilson and Atallah20). High-saturated fat diet is related to oxidative stress(Reference Augusti, Chackery and Jacob21), and has a promoting effect on pancreatic carcinogenesis in azaserine-treated rats(Reference Appel, nan Garderen-Hoetmer and Woutersen22).
In order to delineate the impact of repeatedly heated CO (RCO) use on genotoxicity and/or carcinogenicity, it is important to determine the presence of toxic chemicals which are ingested through repeatedly heated saturated fat-rich diets. Thus, the present study has been designed to investigate the presence of PAH mixture in RCO using HPLC. Furthermore, the potential of RCO consumption to induce genotoxicity, oxidative stress and carcinogenicity in Wistar rats was also investigated.
Materials and methods
Chemicals
Dimethyl sulphoxide, n-hexane, cyclohexane, anhydrous sodium sulphate, dichloromethane, acetonitrile and silica gel were of analytical grade and purity (purchased from Merck Chemical Company, Mumbai, India). PAH standard mix was purchased from Supelco (Bellefonte, PA, USA). Diethylnitrosamine (DEN), 2-acetylaminofluorene, benzo(a)pyrene (B(a)P), γ-glutamyl-4-methoxy-β-naphthylamide, glycylglycine, fast blue BB salt (4-amino-2,5-diethoxybenzanilide diazotated zinc double salt, 4-benzoylamino-2,5-diethoxybenzenediazonion chloride (zinc chloride) salt), ATP disodium salt, sodium-β-glycerophosphate, sodium diethylbarbiturate, glucose-6-phosphate monosodium salt, B(a)P, colchicine, dichlorodihydrofluorescien-diacetate dye, phenazine methosulphate, 2-thiobarbituric acid, 1,1,3,3-tetramethoxy propane, nitroblue tetrazolium, NADPH and NADH were purchased from Sigma Chemical Company (St Louis, MO, USA). Anti-glutathione-S-transferase placental type (Anti-GST-P) and goat anti-rabbit IgG peroxidase secondary antibodies were procured from Calbiochem (Darmstadt, Germany). CO (refined) used in the study was purchased from the local market (Meghdoot Oil Mills Private Limited, Mumbai, India). The rest of the chemicals that were used in the study were of analytical grade and purity, and were procured locally.
Repeatedly heated coconut oil sample preparation
The refined CO was heated (>300°C) above its smoke point (232°C; 450°F) for 30 min, and was cooled to room temperature. For RCO, the process was repeated six times, and the oil sample became viscous dark brown in colour after being repeatedly heated.
HPLC of coconut oil
Extraction and clean-up procedure for PAH was carried out using fresh CO (FCO), single-heated CO (SCO) and RCO according to the protocol of Pandey et al. with slight modifications. Briefly, for the extraction of PAH, 10 g of oil were added to 20 ml of n-hexane and extracted thrice with dimethyl sulphoxide (10 ml) followed by the addition of cold distilled water (4°C) slowly, and re-extraction was done thrice with cyclohexane (solvent was changed for specific extraction of PAH). After washing, it was concentrated and passed through a glass column (20 × 2·2 cm) containing silica gel and anhydrous sodium sulphate. The elution was carried out with a mixture of cyclohexane and dichloromethane, and the mixture was evaporated to dryness. The final residue was dissolved in 1 ml of acetonitrile, and passed through a 0·2 μm filter.
Quantification of the extracted PAH was carried out using an HPLC instrument (Water Associates, Inc., Milford, MA, USA) equipped with a dual pump and Rheo dyne injector with a 20 μl loop. The reversed-phase column used for the analysis was C-18 (E. Merk, Darmstadt, Germany) with a pre-column of the same type. The column was eluted at an ambient temperature (27°C) with 70:30 acetonitrile in water as the solvent at a flow rate of 1·5 ml/min, which was monitored on an UV detector at λ 254 nm. The chromatogram was recorded and processed using Waters Millenium Software (Scientific Equipment Source, Pickering, ON, Canada). The sixteen PAH selection criteria were based on (i) the number of benzene rings in PAH, (ii) PAH generally found in oils following the literature search and (iii) resolution of PAH on HPLC. The peaks of the mixture of PAH in oils were identified by comparing the retention time with that of the standard by using an UV detector for the respective fractions. Quantification of PAH was performed by comparing the integrated peak area with that of the standards(Reference Pandey, Dhawan and Das15).
Animals and treatments
Male Wistar rats (6 weeks of age) were procured from the Indian institute of toxicology research (Lucknow, India) animal breeding colony, maintained under standard conditions and fed ad libitum a synthetic pellet basal diet (Ashirwad, Chandigharh, India) and water. Institutional guidelines for the care and use of animals were followed, and the ethical approval for the experiments was obtained from the institutional animal ethical clearance committee, Indian Institute of Technology Roorkee (IITR). All experimental procedures involving animals were approved by the animal ethical clearance committee, IITR.
Chromosomal aberration and micronuclei induction assays
Rats were randomly divided into two sets of eight groups (Gr) having six rats each. In Gr I, no treatment was given to the rats, whereas in Gr II, B(a)P (100 mg/kg body weight; intraperitoneally) was administered to the rats 24 h before killing. Each rat was administered FCO (0·5 ml) in Gr III, SCO in Gr IV (IVa: 0·25 ml and IVb: 0·5 ml), and RCO in Gr V (Va: 0·1 ml, Vb: 0·25 ml and Vc: 0·5 ml) consecutively by gavage. At the end of 1-week experimental period, rats were killed by cervical dislocation, and bone marrow was collected from their femurs.
Oxidative stress assay
Rats were divided equally into five Gr consisting of ten rats each, where Gr I, II and III rats were given treatments that were similar to those used for the genotoxic assays. SCO (0·5 ml/rat) and RCO (0·5 ml/rat) were given by gavage to other Gr, i.e. Gr IV and V, respectively. After 1 week, rats were killed, and their livers were excised and were homogenised separately in ice-cold PBS (pH 7·4) for enzymatic estimations and reactive oxygen species (ROS) determination.
Medium-term bioassay
A total of seventy-two rats were divided into six Gr. Gr I rats were fed a normal basal diet during the entire study period, i.e.12 weeks, and they served as negative controls. Gr II, III, IV and V rats were given a single dose of DEN (200 mg/kg body weight) intraperitoneally. After 1 week of recovery period, rats of Gr II were fed 0·05 % 2-acetylaminofluorene in a crushed diet, and Gr III, IV and V rats were administered 0·5 ml/rat of FCO, SCO and RCO, respectively, by gavage. Simultaneously, Gr VI rats were administered RCO alone (0·5 ml/rat). Rats were subjected to two-third partial hepatectomy after 3 weeks (Fig. 1). After killing, the livers were excised from each rat, cleaned, weighed and stored at − 80°C until further processing. Tissue sections (10 μm) were cut from the frozen livers using a cryostat microtome (SLEE Medical GmbH, Menz, Germany), and were stained for the histochemical localisation of various markers such as GST-P, γ-glutamyl transpeptidase (GGT), glucose-6-phosphatase (G6Pase), adenosine triphosphatase (ATPase) and alkaline phosphatase (AlkPase).
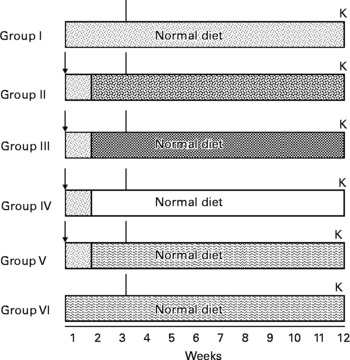
Fig. 1 Treatment schedule for medium-term bioassay. (), Untreated; ( ↓ ), diethylnitrosamine (DEN); (
), 2-acetylaminofluorene (AAF) 0·05 % mixed in crushed diet; (
), fresh coconut oil (FCO) 0·5 ml/animal by gavage; (□), single-heated coconut oil (SCO) 0·5 ml/animal by gavage; (
), repeatedly heated coconut oil (RCO) 0·5 ml/animal by gavage; (∣), partial hepatectomy; K, killed.
Methodology
Chromosomal aberration assay
Colchicine (4 mg/kg of the body weight) was given to the rats 2 h before killing in order to arrest the metaphase stage, and a cytogenetic analysis was carried out(Reference Shukla, Arora and Taneja23). Briefly, the bone marrow was flushed out from both the femurs using Hank's buffered salt solution (pH 7·2) and centrifuged, and the pellet was re-dispersed in a hypotonic solution (0·56 %, w/v) of KCl at 37°C to permit osmotic swelling of cells. Swollen cells were fixed in ice-cold Carnoy's fluid, placed on slides and stained with a phosphate-buffered 5 % Giemsa solution. A total of seventy-five well-spread metaphase plates per rat in each Gr were analysed for chromosomal aberrations at a magnification of 100 × , and the mitotic index was calculated from a scan of 1000 cells per rat and classified as breaks, fragments and exchanges. The incidence of aberrant cells was expressed as the percentage of damaged cells (aberrant metaphases).
Micronuclei induction assay
The frequency of micronucleated polychromatic erythrocytes was evaluated using a modified protocol of Shukla et al. (Reference Shukla, Arora and Taneja23). The bone marrow was flushed from both the femurs using Hank's buffered salt solution, 1 % (w/v) bovine serum albumin and 0·15 % (w/v) EDTA (pH 7·2). Evenly spread bone marrow smears were stained using the May–Grunwald and Giemsa stain. A total of 1000 polychromatic erythrocytes were scored for each treated and control Gr.
Biochemical estimations of antioxidant enzymes
The protein content of the tissue was determined by the method of Lowry et al. (Reference Lowry, Rosebrough and Farr24) using bovine serum albumin as the standard. Cu/Zn superoxide dismutase activity was analysed as per the protocol of Kakkar et al. (Reference Kakkar, Das and Viswanathan25), and was expressed as specific activity (units/min per mg protein). The activity of catalase was analysed according to the method of Sinha(Reference Sinha26) using hydrogen peroxide as the substrate. The enzyme activity was measured following the disappearance of hydrogen peroxide, and expressed as μmol of hydrogen peroxide consumed/min per mg of protein. Lipid peroxidation (LPO) was analysed by the method of Ohkawa et al. (Reference Ohkawa, Ohisi and Yagi27), and peroxides were expressed as nmol of thiobarbituric acid-reactive substance/h per mg of tissue protein using 1,1,3,3-tetramethoxy propane as the standard.
Reactive oxygen species generation
ROS production was monitored by flow cytometry (BD-LSR II, San Jose, CA, USA) using dichlorodihydrofluorescien-diacetate dye(Reference Esposti and McLennan28). The fluorescence increased due to the hydrolysis of dichlorodihydrofluorescien-diacetate to dichlorodihydrofluorescien by some non-specific cellular esterases, and its subsequent oxidation by peroxides was measured and expressed in terms of mean fluorescence intensity using the software ‘CellQuest’.
Medium-term bioassay
Immunohistochemical localisation of GST-P activity was performed as per the protocol of Tatematsu et al. (Reference Tatematsu, Mera and Inoue29). The exogenous peroxidase activity in fresh sections of liver was quenched with methanol: hydrogen peroxide solution in dark, and the non-specific binding was blocked using normal goat serum. The sections were incubated with the primary anti-GST-P antibody (1:300), and then with the respective secondary antibody. The colour was developed using 3-amino-9-ethylcarbazole and counterstained with haematoxylin. The histochemical activities of GGT, G6Pase, ATPase and AlkPase in rat liver were detected by the method of Shukla & Arora(Reference Shukla and Arora30). Briefly, to detect GGT activity, the sections were incubated in a medium containing γ-glumatyl-4-methoxy-β-naphthlylamide dissolved in dimethyl sulphoxide, glycylglycine and fast blue BB salt, and were transferred into a copper sulphate solution, washed and mounted. To detect G6Pase activity, sections were incubated in a medium containing lead nitrate and glucose-6-phosphate at 37°C for 30 min, and were developed in yellow ammonium sulphide. The brownish black deposits of lead sulphide indicated the active site of G6Pase activity. To detect ATPase activity, the sections were incubated in a medium containing lead nitrate, magnesium chloride and ATP for 60 min at room temperature, and were developed in yellow ammonium sulphide. Brown precipitates indicated the site of enzyme activity. For the localisation of AlkPase activity, the sections were incubated in a freshly prepared medium containing sodium β-glycerophosphate, sodium diethylbarbiturate, calcium chloride and magnesium sulphate. After incubation, sections were transferred to cobalt nitrate solution and developed in yellow ammonium sulphide. The black deposits of cobalt sulphide indicated the site of enzyme activity.
The activity of biomarker enzymes was observed with reference to the area and count of altered hepatic foci (AHF) in each Gr using Leica QWin500 image analysis software (Leica Mikrosysteme Vertrieb GmbH, Wetzlar, Germany). The image analysis was performed for each slide in triplicates with at least ten fields in each slide. The foci were scored only if their diameter was >0·20 mm.
Statistical analysis
The statistical analysis for different parameters between different Gr was evaluated using Student's t test; P < 0·05 was considered significant for all the assays.
Results
Presence and amounts of polycyclic aromatic hydrocarbons in repeatedly heated coconut oil
On the basis of their molecular weight, carcinogenicity and ring sizes, PAH have been categorised into two classes: ‘light PAH’, such as naphthalene, acenaphthylene, fluorene+acenaphthene, phenanthrene, anthracene, fluoranthene and pyrene, and ‘heavy PAH’, such as benzo(a)anthracene+chrysene, benzo(k)fluoranthene, benzo(b)fluoranthene, B(a)P, dibenzo(a, h)anthracene, indeno(1,2,3cd) pyrene and benzo(g, h, i)perylene(Reference Moret, Purcaro and Conte31). Higher amount of PAH was present in RCO (160·0 μg/kg) than in FCO (30·2 μg/kg) and SCO (66·3 μg/kg) (Table 1 and Fig. 2). The amount of light PAH was 19·3 μg/kg (64 % of the total PAH) in FCO extract, 37·9 μg/kg (57 % of the total PAH) in SCO extract and 67·3 μg/kg (42 % of the total PAH) in RCO extract. RCO showed an increase in the concentrations of naphthalene (9-fold), fluoranthene (16-fold) and pyrene (21-fold) among the light PAH than FCO. The quantity of heavy PAH in the extracts prepared from FCO, SCO and RCO was 10·9 μg/kg (36 % of the total PAH), 28·4 μg/kg (43 % of the total PAH) and 92·7 μg/kg (58 % of the total PAH), respectively. Apparently, out of the total content of PAH in the oil extracts, the amount of heavy PAH was more in RCO than in FCO and SCO. RCO showed an increase in the concentrations of benzo(a)anthracene+chrysene (9-fold), benzo(k)fluoranthene (10-fold), benzo(b)fluoranthene (11-fold), B(a)P (14-fold), dibenzo(a, h)anthracene and indeno(1,2,3cd)pyrene+benzo(g, h, i)perylene (6-fold) compared with FCO (Fig. 2(c)).
Table 1 The amount (μg/kg) of polyaromatic hydrocarbons (PAH) in fresh (FCO), single-heated (SCO) and repeatedly heated coconut oil (RCO) samples
(Mean values with their standard errors of three independent set of analyses)
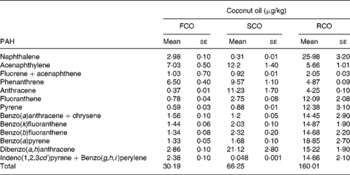
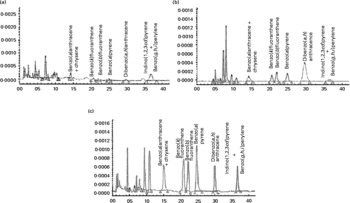
Fig. 2 HPLC chromatograms (autoscaled) showing the presence of polyaromatic hydrocarbons in coconut oil: (a) fresh coconut oil, (b) single-heated coconut oil and (c) repeatedly heated coconut oil.
Effects of repeatedly heated coconut oil repeatedly heated coconut oil on induction of micronuclei and chromosomal aberrations
Administration of RCO caused a higher incidence of micronuclei and chromosomal aberrations (Table 2 and Figs. 3 and 4) in rats. An induction in micronuclei of up to 2·3-fold was found in the positive control (B(a)P administered) Gr. A significant induction in the percentage of aberrant cells of up to 2·2-, 2·3- and 2·4-fold was observed in the RCO-administered Gr Va, b and c, respectively. Mitotic index was also found to decrease up to 1·6-fold in Gr Va, 2·3-fold in Gr Vb and 2·4-fold in Gr Vc. A dose-dependent increase (P < 0·05) in the incidence of micronucleated polychromatic erythrocytes/1000 polychromatic erythrocyte was recorded in the RCO-administered rats (Table 2). SCO administration increased aberration, micronuclei induction and cytotoxicity up to a marked level compared with the FCO treatment and controls (P < 0·05).
Table 2 Effects of repeatedly heated coconut oil treatment on mitotic index, chromosomal aberration and micronuclei induction in Wistar rats
(Mean values with their standard errors of ten rats)

Gr, groups; MNCPE, micronucleated polychromatic erythrocytes; PCE, polychromatic erythrocyte; B(a)P, benzo(a)pyrene.
Mean values were significantly different for induction and suppression over untreated Gr I: *P < 0·05, **P < 0·05.
† Gr I, untreated; Gr II, B(a)P (100 mg/kg body weight) treated; Gr III, fresh coconut oil (0·5 ml/rat) treated; Gr IV, single-heated coconut oil (IVa: 0·25 ml/rat and IVb: 0·5 ml/rat) treated; Gr V, repeatedly heated coconut oil (Va: 0·1 ml/rat, Vb: 0·25 ml/rat and IVc: 0·5 ml/rat) treated.
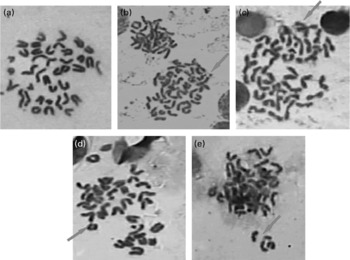
Fig. 3 Representative pictures (100 × ) showing chromosomal aberrations induced by repeatedly heated coconut oil (0·5 ml/rat) in bone marrow of Wistar rats (a) no aberration; (b) exchange; (c) break; (d) ring and (e) fragment.
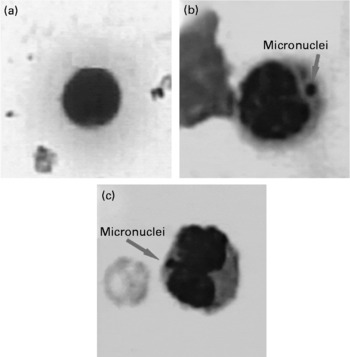
Fig. 4 Representative pictures showing micronuclei (100 × ) in (a) untreated; (b) benzo(a)pyrene-treated (100 mg/kg body weight) and (c) repeatedly heated coconut oil-treated (0·5 ml/rat) groups.
Effects of repeatedly heated coconut oil on induction of oxidative stress
A significant decrease in the levels of antioxidant enzymes catalase (65·1 %) and superoxide dismutase (45·6 %) were found in B(a)P-administered Gr II than in Gr I (Table 3). The activity of catalase (55·0 %) and superoxide dismutase (28·0 %) also declined in RCO-fed rats (P < 0·05). A considerable induction in LPO levels was observed in B(a)P (80 %)- and RCO (40 %)-administered rats compared with the untreated controls (Table 3). However, in FCO (Gr III)- and SCO (Gr IV)-treated Gr, no significant changes in the studied parameters were observed (P>0·05).
Table 3 Effects of repeatedly heated coconut oil treatment on levels of antioxidant enzymes and lipid peroxidation (LPO) in Wistar rats
(Mean values with their standard errors of ten rats)

Gr, groups; TBARS, thiobarbituric acid-reactive substance.
Mean values significantly decrease in the level of antioxidant enzymes over untreated Gr I and significantly increase in LPO of rats over untreated Gr I: *P < 0·05, **P < 0·05.
† Numbers given in the parentheses indicate the percentage change over untreated Gr I.
‡ Gr I, untreated; Gr II, B(a)P (100 mg/kg body weight) treated; Gr III, fresh coconut oil (0·5 ml/rat) treated; Gr IV, single-heated coconut oil (0·5 ml/rat) treated; Gr V, repeatedly heated coconut oil (0·5 ml/rat) treated.
The determination of intracellular ROS generation in terms of the mean fluorescence intensity of dichlorodihydrofluorescein-diacetate dye indicated 94·66 (se 1·5) in the untreated Gr, which significantly increased up to 176·68 (se 6·6) in the B(a)P-fed Gr, 147·29 (se 10·2) in the RCO-fed Gr and 129·38 (se 3·4) in the SCO-fed Gr.
Effects of repeatedly heated coconut oil on induction of altered hepatic foci
Rats administered DEN+2-acetylaminofluorene (Gr II) exhibited a significant (P < 0·05) decrease in both body weight (135·5 (se 21·5) v. control 210·0 (se 21·5)) and relative liver weight (2·84 (se 0·3) v. control 3·34 (se 0·4)) compared with Gr I rats. RCO along with DEN also decreased relative liver weight (3·00 (se 0·4); P < 0·05), whereas no change in body weight was observed. FCO and SCO treatments had no effects on the body weight and liver weight of rats when compared with the treatment given to Gr I rats (P>0·05).
The results revealed significant changes in positive (increase in GST-P and GGT) and negative biomarker (decrease in AlkPase, ATPase and G6Pase) levels in Gr given DEN+2-acetylaminofluorene (Gr II), DEN+RCO (Gr V) and RCO alone (Gr VI) with respect to the untreated Gr, Gr I (Tables 4 and 5 and Fig. 5; P < 0·05). No alterations were observed in any positive or negative biomarkers by oral administration of either FCO (Gr III) or SCO (Gr IV) compared with the untreated controls (P>0·05).
Table 4 Altered hepatic foci-inducing effects of repeatedly heated coconut oil treatment in Wistar rats in terms of the percentage area (foci/mm2)
(Mean values with their standard errors of ten rats)

Gr, groups; DEN, diethylnitrosamine; 2-AAF, 2-acetylaminofluorene; FCO, fresh coconut oil, SCO, single-heated coconut oil; RCO, repeatedly heated coconut oil.
* Mean values were significantly different in induction ( ↑ ) in the level of positive biomarkers with respect to Gr I (P < 0·05).
† Numbers given in the parentheses indicate the percentage change over to Gr I.
‡ Gr I, untreated; Gr II, DEN (200 mg/kg body weight)+2-AAF (0·05 % in crushed diet) treated; Gr III, DEN (200 mg/kg body weight)+FCO (0·5 ml/rat) treated; Gr IV, DEN (200 mg/kg body weight)+SCO (0·5 ml/rat) treated; Gr V, DEN (200 mg/kg body weight)+RCO (0·5 ml/rat) treated; Gr VI, RCO (0·5 ml/rat) treated.
§ The symbol ↓ represents significant suppression in the level of negative biomarkers.
Table 5 Altered hepatic foci-inducing effects of repeatedly heated coconut oil treatment in Wistar rats in terms of the count of foci/mm2
(Mean values with their standard errors of ten rats)

Gr, groups; DEN, diethylnitrosamine; 2-AAF, 2-acetylaminofluorene; FCO, fresh coconut oil; SCO, single-heated coconut oil; RCO, repeatedly heated coconut oil.
* Mean values were significantly different in induction in the level of positive biomarkers with respect to Gr I (P < 0·05).
† Numbers given in the parentheses indicate the percentage change over to Gr I.
‡ Gr I, untreated; Gr II, DEN (200 mg/kg body weight)+2-AAF (0·05 % in crushed diet) treated; Gr III, DEN (200 mg/kg body weight)+FCO (0·5 ml/rat) treated; Gr IV, DEN (200 mg/kg body weight)+SCO (0·5 ml/rat) treated; Gr V, DEN (200 mg/kg body weight)+RCO (0·5 ml/rat) treated; Gr VI, RCO (0·5 ml/rat) treated.
§ Significant suppression in the level of negative biomarkers are represented.
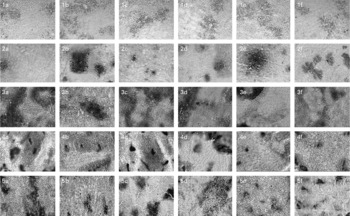
Fig. 5 Representative pictures (10 × ) for cellular localisation of the expression of liver-specific enzyme markers showing (1) γ-glutamyl transpeptidase, (2) glutathione-S-transferase, (3) ATP, (4) glucose-6-phosphatase, (5) alkaline phosphatase, where (1a–5a) represent untreated group; (1b–5b) represent diethylnitrosamine (200 mg/kg body weight)+2-acetylaminofluorene (0·05 % in crushed diet)-treated group; (1c–5c) represent diethylnitrosamine (200 mg/kg body weight)+fresh coconut oil (0·5 ml/rat)-treated group; (1d–5d) represent diethylnitrosamine (200 mg/kg body weight)+single-heated coconut oil (0·5 ml/rat)-treated group; (1e–5e) represent diethylnitrosamine (200 mg/kg body weight)+repeatedly heated coconut oil (RCO; 0·5 ml/rat)-treated group; (1f–5f) represent RCO (0·5 ml/rat)-treated group.
Discussion
Food that we eat may contain carcinogenic contaminants, or may become a mutagen itself after the transformation of some of its components(Reference Uauy and Solomons32). To date, many dietary constituents have been studied for their carcinogenic or mutagenic potential(Reference Taghavi and Yazdi3, Reference Chiang, Pei-Fen and Ying11, Reference Sauer, Blask and Dauchy33). Relationship between dietary use of vegetable fats and risk of cancers has long been known, but studies exploring the mechanism for this connection are ambiguous. In this context, the present study was conducted to identify and quantify the contaminants generated in CO during the process of repeated heating to the temperatures usually used for cooking. There are reports regarding the formation of carcinogenic compounds by the heat processing of vegetable oils(Reference Chiang, Pei-Fen and Ying11, Reference Wu, Chiang and Wang12). These compounds may form DNA adducts, which have been proposed as predictive biomarkers of human cancers(Reference Schaffer and Milner34). Results of the study revealed the presence of a higher amount of total PAH in RCO (six times heated) than in SCO and FCO (Table 1). Pandey et al. (Reference Pandey, Dhawan and Das15) also reported that repeated heating of edible oil at high temperatures generates greater amounts of PAH, which are dependent on the number of cycles used for heating. Moreover, RCO contained a higher amount of heavy PAH of the total PAH than FCO and SCO. Heavy PAH are reported to have significant carcinogenic and mutagenic potential(Reference Marsili, Caruso and Fossi35).
As the amount of PAH was high in RCO, it is assumed that its dietary use may result in risk of genotoxicity and carcinogenicity. The assays performed to detect both chromosomal aberration and micronuclei induction in the bone morrow are considered to be able to detect early effects of genotoxicity following the exposure to mutagens and carcinogens(Reference Shukla, Arora and Taneja23). By using these two assays in the present study, RCO was found to enhance the incidence of aberrant cells, including breaks, fragments, exchanges and multiple chromosomal damages and micronuclei in a dose-dependent manner (Table 2). Chromosomal aberration and micronuclei induction by B(a)P(Reference Shukla, Arora and Taneja23) and heated cooking oils(Reference Isidori and Parrella36) are well documented. The results of the present study suggest that RCO may contain a high amount of B(a)P (Table 1).
The prevention of oxidation is an essential process as decreased antioxidant levels may lead to cytotoxicity, mutagenicity and carcinogenicity(Reference Mates37, Reference Narchonai, Shila and Panneerselvam38). There is evidence linking the metabolism of PAH with the generation of ROS and thus oxidative stress(Reference Camus, Birkely and Jones39). Cooking oil fumes were found to induce DNA damage via ROS production(Reference Dung, Wu and Yen8). Our results revealed a significant (P < 0·05) decrease in the levels of antioxidant enzymes (viz. catalase and superoxide dismutase) and increase in the levels of LPO and ROS following RCO administration (Table 3). Accordingly, the decrease in the levels of antioxidant enzymes has been observed earlier in rats fed heated and fried oil(Reference Narasimhamurthy and Raina40). Altered levels of antioxidant enzymes are evident in cancers(Reference Narchonai, Shila and Panneerselvam38). From the present set of observations, it has been concluded that RCO has the potential to cause oxidative imbalance by decreasing antioxidant enzyme levels and increasing ROS and LPO, which in turn may cause susceptibility towards cancer development(Reference Dung, Wu and Yen8). Ip & Sinha(Reference Ip and Sinha41) reported that saturated fat induces LPO. A study done by Udilova et al. (Reference Udilova, Jurek and Marian42) suggested that the peroxidation of biomembranes by hydroperoxides from heated oils may contribute to enhanced risk of cancers. In another study, 1, N(6)-ethenodeoxyadenosine, which is determined as a marker for DNA damage caused by LPO products and a marker for oxidative stress, formed the highest adducts in the CO diet(Reference Eder, Wacker and Lutz43).
Induction of preneoplastic lesions is among the events leading to liver tumour development, and has been used to assess the carcinogenic/anticarcinogenic potential of various agents(Reference Shukla and Arora30). A number of genotoxic responses have been reported in fresh or heat-treated foodstuffs, which are also reported to be carcinogenic in rodent bioassays. Earlier, we have shown that heated mustard oil induces AHF significantly(Reference Shukla and Arora30). In the present study, investigations were performed for the evaluation of AHF-developing potential of RCO. A significant enhancement in the expression of positive biomarker enzymes (GGT and GST-P) and a reduction in negative markers (AlkPase, ATPase and G6Pase) were observed in the rats administered DEN+RCO and RCO alone compared with the FCO- and SCO-treated Gr (Tables 4 and 5). GST-P is used as a diagnostic marker for hepatocellular carcinoma, and is also used for the detection of other chronic liver diseases(Reference Yusof, Yan and Hussain44). Another study suggested that a deficiency in canalicular ATPase and G6Pase provides the best marker for the larger foci and nodules, and thus can be used to identify preneoplastic changes in the liver(Reference Glauert, Beer and Rao45). Aruna et al. (Reference Aruna, Rukkumani and Varma46) suggested that initiation of hepatocarcinogenesis is influenced by thermally oxidised vegetable oil. The AHF-inducing activity of RCO in DEN-initiated rats may be attributed to its SFA. Repeatedly used frying oils when administered to rats have been shown to produce indications of cellular damage to the liver and kidneys(Reference Hageman, Verhagen and Schutte47). RCO leads to the induction of oxidative stress, which in turn inhibits the activity of enzymes such as G6Pase and ATPase within the cells(Reference Wu and Yen13). Increase in ROS production is related to epigenetic toxicity(Reference Arbillaga, Azqueta and van Delft48), and also accounts for GST-P induction in hepatocytes(Reference Kimihiko and Ichiro49). Since tumour initiation and promotion involve genetic and epigenetic pathways, respectively, it seems like RCO (Table 1) having more amounts of carcinogenic PAH and inducing AHF alone as well as with DEN works both as an initiator and as a promoter.
In conclusion, the presence of a high amount of carcinogenic PAH in RCO was responsible for oxidative stress via enhancement of ROS generation and LPO, thus leading to the formation of preneoplastic lesions in rat liver. Therefore, dietary consumption of RCO exhibits the possible risk of carcinogenicity and genotoxicity.
Acknowledgements
The authors thank all their colleagues who were involved in the study for their excellent assistance. All authors participated in the development of the study concept and design; S. S. and M. S. were responsible for the execution of the study; K. B. was responsible for the flow cytometry work; S. S. and A. M. S. were responsible for the chemical analysis of oil samples; J. G. and Y. S. were responsible for the Image analysis; all authors were involved in the data interpretation; S. S. wrote the draft of the manuscript; M. S., A. M. S., J. G., K. B. and Y. S. reviewed and revised the manuscript. The authors thank the Director, Indian Institute of Toxicology Research, Lucknow, for his keen interest in the study. They are also thankful to the analytical toxicology section of IITR for their technical support and assistance with HPLC analysis. The authors are thankful to CSIR, New Delhi, for funding this work from Task force project NWP-17. No conflicts of interest are declared by the authors.