The trace element iodine is essential for the production of thyroid hormones tri-iodothyronine and thyroxine (T4) in humans and animals(Reference Zimmermann, Jooste and Pandav1). Beside iodised salt, cows’ milk and dairy products have become major sources of nutritional iodine in many industrialised countries over the last decades, with an estimated contribution of approximately 25–70 % to the daily iodine intake(Reference Haldimann, Alt and Blanc2–Reference Dahl, Johansson and Julshamn7). However, iodine concentration in milk was found to vary across production systems and seasons, with a 70 % median increase from organic to conventional and a 90 % median increase from summer to winter(Reference van der Reijden, Galetti and Hulmann8). This variation is mainly explained by iodine concentration in the feed, the use of iodine-containing products for teat disinfection practices and by milk treatment(Reference van der Reijden, Galetti and Hulmann8–Reference van der Reijden, Zimmermann and Galetti11). This makes milk, and consequently dairy products, an unpredictable dietary source of iodine. If stable iodine concentrations in milk and dairy products could be achieved, the contribution of these products to human iodine nutrition could be optimised, and both iodine deficiency and excess could be avoided. Both conditions are regarded as a public health concern, with nineteen and ten countries worldwide showing inadequate and excessive intakes in 2017, respectively(Reference Eastman, Zimmermann, De Groot, Chrousos and Dungan12).
In dairy cow nutrition, iodine requirement is estimated to be met by 0·5 mg/kg dietary DM(13), which is ten times lower than the maximum authorised concentration of 5 mg/kg of complete dairy cow diet (assuming 88 % DM) according to European Union (EU) regulation(14). However, the European Food Safety Authority (EFSA) recently proposed a reduced maximum level of 2 mg iodine/kg of complete dairy cow diet (88 % DM)(15) following the concern that the authorised maximum iodine level in feed could lead to excessive iodine concentrations in milk and dairy products, posing a risk of exceeding the upper limit for adults and adolescents. However, concerns were also raised that the proposed lower maximum level of iodine in animal feed would potentially increase the risk of iodine deficiency in already vulnerable human populations(Reference Bath and Rayman16).
Previous studies have reported a positive relationship between feed and milk iodine in the range of 0–10 mg iodine/kg of feed(Reference Flachowsky, Franke and Meyer9, Reference Schone, Leiterer and Lebzien17–Reference Borucki Castro, Berthiaume and Robichaud25); however, this was based on limited data at supplementation levels <2 mg iodine/kg of feed. The cow diets investigated were mainly based on maize silage and concentrate, with grass-based feeds making up proportions of <25 % of DM. The presence of known (e.g. glucosinolates) and unknown iodine antagonists in feeds, which can affect iodine transfer into milk, may differ between feeds. Thus, there is a need to quantify the relationship of feed with milk iodine also from cows fed grass-based diets. These diets, characterised by low concentrate proportions, are very common in grassland-dominated areas of a number of countries within and outside of Europe(Reference Dillon, Crosse and Stakelum26, Reference Reidy and Ineichen27).
Although dairy products such as yogurt and cheese are also important contributors of iodine in the human diet, their iodine concentration has been rarely measured. There is especially a gap in knowledge concerning the effect of processing (pasteurisation, sterilisation, skimming, spray-drying) on milk iodine concentration (MIC). It is thought that inorganic forms of iodine, making most of the iodine in milk, sublimate during heat treatment and result in a decreased iodine concentration in heat-treated milk, but available results concerning heat treatment are controversial(Reference Wheeler, Fleet and Ashley28–Reference Norouzian31). The evidence on the effect of skimming whole milk on iodine concentrations is limited to one negative study(Reference Wheeler, Fleet and Ashley28). Researchers investigated the influence of fat concentration on milk, but did so only by comparing retail milks with different fat concentrations(Reference Arrizabalaga, Jalón and Espada6, Reference Soriguer, Gutierrez-Repiso and Gonzalez-Romero32–Reference Rasmussen, Carlé and Jørgensen34). Iodine in cheese originates not only from the milk used for production, but can also result from the addition of iodised salt during manufacturing into the milk or curd, on the cheese surface or into brine. This is intended to improve taste, increase whey drainage, promote rind formation or contribute to bacteriological selection. However, the use of iodised salt in Swiss cheese production has progressively been replaced by the use of non-iodised salt due to the Swiss food information regulation stating that cheese containing iodised salt must be labelled as such, whereas no label is required for cheese containing non-iodised salt(3, 35).
Therefore, the objectives of the present study were: (1) to evaluate the dose–response relationship between increasing feed iodine supplementation, starting from a low level, with milk iodine in dairy cows fed a diet based on grassland-based feeds (experiment 1); (2) to capture the losses of iodine during cheese and yogurt manufacturing from cows’ milk having variable iodine concentrations and without iodised salt addition during the production (experiment 2); (3) to investigate the effect of heat treatment and skimming on iodine concentration (experiment 3); and finally (4) to calculate the contribution of milk and dairy products to the Swiss adults’ dietary iodine intake at different levels of iodine supplementation into the dairy cows’ diet.
Materials and methods
Experiment 1: iodine transfer from feed to cows’ milk
The experiment took place from November to December 2015 at the Agroscope research farm in Posieux, Switzerland. The protocol was approved by the Swiss Office for Food Safety and Veterinary Affairs (Authorisation 2015_48_FR) and conducted in accordance with the Swiss Ordinance on Animal Protection and the Ordinance on Animal Experimentation. Twenty-five lactating Red Holstein and Holstein cows (body weight (mean ± sd) 644 ± 58 kg; parities 2·1 ± 1·1; days in milk 60 ± 23; daily milk yield 29·3 ± 6·6 kg) were used. They were first provided a diet without supplemental iodine during a 21-d adaptation period to minimise the effects of previous iodine intake. At the end of the adaptation period, they were blocked by parity, days in milk and milk yield. Each cow within five blocks was then randomly assigned to be supplemented with 0, 0·5, 0·7, 1 or 2 mg iodine/kg DM of feed over a period of 21 d (day 0 to day 21). The upper dose of 2 mg/kg DM was chosen to correspond to the maximum supplemental iodine level as recommended by the EFSA(15). The dose of 0·5 mg iodine/kg DM corresponds to the recommended minimal iodine supply for lactating dairy cows(13). A dose of 0·7 mg iodine/kg DM was chosen as it was estimated to result in an MIC contributing 30–40 % of the adults’ RDA for iodine (150 μg/d)(Reference Haldimann, Alt and Blanc2, 3, 36) based on existing dose–response equations with other cow diets(Reference Flachowsky, Franke and Meyer9, Reference Franke, Meyer and Wagner18) and an estimated milk consumption of 290 ml/d(Reference Haldimann, Alt and Blanc2).
The basal cow diet was formulated to cover the requirements for all nutrients, minerals and trace elements, except iodine, according to the Swiss feeding recommendations and considering 650 kg body weight and 35 kg/d milk yield(37). The diet consisted of a mixed ration (MR) of (based on DM) 32 % grass silage, 32 % hay, 19 % maize silage and 17 % dehydrated lucerne, which was prepared daily. Two concentrates were produced in the Agroscope experimental feed mill to contain 0 and 11·15 mg iodine/kg DM, respectively, which were proportionally fed to the cows to reach the respective target supplementation levels (Table 1). Both concentrates consisted of (based on fresh matter): 35·15 % barley, 24·4 % maize, 11·852 % maize gluten, 15 % soyabean meal, 3 % beet molasses, 2·8 % calcium carbonate, 2·2 % iodine-free sodium chloride, 1 % magnesium oxide, 0·5 % monocalcium phosphate, 0·35 % diluted premix and 0·6 % colour tracer. The diluted premix consisted of 90 % maize gluten and 10 % iodine-free trace mineral and vitamin premix (Vital AG). The premix was either supplemented with 418·9 mg/kg KI (Sigma-Aldrich) or remained non-supplemented.
Table 1. Nutrient composition of the unfortified concentrate, the fortified concentrate and the mixed ration fed in experiment 1, and proportions of the two concentrates fed to the five treatment groups over the 21-d experimental period*
(Mean values and standard deviations, single values, and proportions)

PDIE, protein digestible in the small intestine calculated from its rumen-available energy content; PDIN, protein digestible in the small intestine calculated from its non-degradable N and degradable N contents.
* Data are presented as means and standard deviations in the case of multiple measurements or as actual values in the case of a single measurement. Net energy for lactation, PDIE and PDIN are calculated values(Reference Schlegel, Wyss and Arrigo39).
† For thiocyanate: n 1 for unfortified concentrate; n 1 for fortified concentrate; single analysis. For iodine: n 3 for unfortified concentrate; n 7 for fortified concentrate (sd ± 0·92 mg/kg); triplicate analysis. For all other parameters: n 3 for unfortified concentrate; n 1 for fortified concentrate; duplicate analysis. One sample is a representative mixture of concentrates over the whole experimental period.
‡ For all parameters: n 6; duplicate analysis. For thiocyanate: single analysis. For iodine: triplicate analysis. One sample of mixed ration was analysed from each experimental week.
During the experiment, cows were kept in a free-stall barn with permanent access to water and an enclosed yard outside. MR was offered ad libitum in feeding troughs connected to electronic balances (Insentec B.V.) that recorded every cow visit and its individual MR intake. The two concentrates were supplied at defined amounts per cow by a transponder feeding station (Arnold Bertschy AG). During the first 2 weeks of adaptation, a fixed amount of concentrate (4 kg/d per animal) was offered. From then on, in order to maintain the target iodine concentration in each cow’s diet, the daily allowance of concentrate was adapted on a weekly basis according to the individual average MR intake measured on the previous 7 d.
Milking took place twice daily at 04.45 and 16.00 hours in a milking parlour. Milk yield was recorded with automatic milk counters. After 3 weeks of adaptation, individual milk samples were collected from two consecutive milking events (i.e. evening and morning) on days 0, 3, 7, 14 and 21 using a device that continuously collected milk proportionate to milk flow during milking to get a representative sample of the whole milking event. Morning and evening milk samples from days 0, 3, 7 and 14 were pooled proportionally to milk yield by animal and day, whereas morning and evening milk samples from day 21 were kept separate to detect time-of-day variations. Milk samples were frozen at –20°C until analysis. To avoid exogenous iodine contamination, an iodine-free teat dip (Mammo-Film+; Multiforsa AG) was used after each milking, this already in the adaptation period, and sampling material was dishwasher-cleaned using an iodine-free cleaning product.
Blood was sampled from the jugular vein on days 0 and 21 at 09.00 hours in 9-ml lithium heparin-coated vacutainers (Greiner Bio-One). Samples were kept on ice until centrifugation (3000 g for 15 min) and the retrieved plasma was stored at –20°C until analysis.
Samples of unfortified and fortified concentrates were collected daily from the transponder feeding stations and kept at –20°C until analysis. Individual MR components (grass silage, hay, maize silage and dehydrated lucerne) and MR samples were collected once weekly and lyophilised (Christ-Delta 1-24 LSD; Martin Christ Gefriertrocknungsanlagen GmbH) for 72 h. Samples of each feed item were pooled to form a representative sample for each component and ground using a 1-mm screen (Brabender Mill; Brabender). All samples were stored in sealed jars at room temperature until analysis. Prior to the experiment start, water was sampled from various taps in the barn using 15-ml polypropylene tubes with screw caps (Semadeni) and kept at −20°C until analysis.
Experiment 2: iodine transfer from milk to dairy products
The experiment took place from February to June 2016 on the experimental farm and cheese factory of the Institut Agricole Régional, Aosta, Italy. All animal-related procedures were in compliance with EU Directive 2010/63/EU. Sixty-two lactating Aosta Red Pied cows (mean baseline herd milk yield (mean ± sd) 17·8 ± 4·6 kg/d) were housed in a tie-stall and fed with hay and a concentrate without any supplemental iodine. After an iodine depletion period of 14 d, each cow received 15·7 ± 0·4 g barley patty (1:1·05 barley flour to water ratio, analysed native iodine concentration of 3 μg/kg DM, 47·3 % DM) twice per d, after morning and evening milking. The barley patty was fortified with 0, 325 ± 5, 461 ± 7 and 911 ± 10 mg iodine/kg as KI (Sigma-Aldrich) during weeks 1–2, 3–4, 5–6 and 7–8, respectively. Based on a daily analysis, patties provided 0·0, 10·2 ± 0·4, 14·5 ± 0·3 and 28·7 ± 0·5 mg iodine/d to each cow, respectively, and assuming a DM intake of 14·5 kg/d per cow, diets were supplemented with approximately 0, 0·7, 1, and 2 mg iodine/kg of diet DM, respectively.
During the last 3 d of each 2-week period, morning and evening milk was collected for further processing. Five dairy products were manufactured during each period. These included: (1) Valle d’Aosta Fontina Protected Designation of Origin (PDO), a semi-hard cheese, which is produced from raw whole milk from a single milking, processed within a few hours from milking and ripened for at least 90 d; (2) Toma, another semi-hard cheese, which is produced from raw morning milk with a 1:1 addition of partially skimmed evening milk and ripened for at least 60 d; (3) Tomino, a fresh and small-sized cheese, which is produced from pasteurised whole milk with added salt (about 550 g per 100 litres milk) and the curd of which is cut to grains of about 6 cm diameter and drained in mould for about 1 d; (4) Reblec, a mid-sized fresh cheese, which is produced from pasteurised whole milk, where the curd is drained in mould for about 10 h and the cheese is frequently turned over; and (5) plain white yogurt produced from pasteurised milk.
In each 2-week period, four independent batches of Valle d’Aosta Fontina PDO and Toma, two independent batches of Reblec, one batch of Tomino, and one batch of yogurt were produced. For each of the cheese batches, milk, whey and curd after whey drainage were sampled. In addition, for each batch of semi-hard cheese, two cheese wheels were sampled by core drilling 24 h after pressing in mould, and after cellar-ripening for 14, 28 and 56 d (Toma) and for 14, 42 and 84 d (Valle d’Aosta Fontina PDO). Yogurt was only sampled as an end-product complemented by samples of the corresponding milk for its production. All samples were stored at −20°C until analysis.
Like in the first experiment, no iodine-containing agents were applied for cleaning and disinfection and only iodine-free salt was used for salting and brining of cheeses. Accidentally, during Tomino production in the zero supplementation period, about 250 g iodised salt (32 parts per million), instead of non-iodised salt, were added into about 60 litres of milk. The iodine concentration in the corresponding end-product was treated as outlier and excluded from analysis.
Experiment 3: milk heat treatment and skimming
For the heat treatment experiment, high- and low-iodine milk were produced at AgroVet-Strickhof, Lindau, Switzerland, by either feeding cows a supplemented diet containing 99 mg KI/d (5 mg iodine/kg of DM intake assuming a DM intake of 15 kg/d) for 14 d or no supplemental iodine. After 2 weeks, evening milk and morning milk of the following day were collected and cooled down to <6°C within 1 h after milking. Evening and morning milk were pooled and subsequently pasteurised and homogenised (Bischof Anlagenbau AG). Aliquots of about 330 ml were filled into 500-ml high-density polyethylene bottles (Semadeni) and frozen at –20°C. Duplicate samples of high- and low-iodine milks both prior to and post-pasteurisation and homogenisation were analysed for iodine speciation (i.e. quantification of iodine as iodide and iodate and an unknown organic iodine fraction) and for total iodine.
For the skimming experiment, untreated raw milk samples (1 litre) were collected from farms in the cantons of Zurich (n 6) and Fribourg (n 13), Switzerland. This was accomplished within 2 weeks in January/February 2017 in order to exclude potential seasonal vegetation influences on MIC. Milk was collected from bulk tanks or vending machines from the last 24 h to guarantee optimal quality for the skimming process. The raw milk samples were stored at 4°C until skimming, which took place latest 36 h after milk collection. After measuring fat concentration, about 40 ml of each sample was transferred into 50-ml polypropylene tubes with screw caps (Sarstedt). The tube was placed in a water bath on a stirring hot plate (Fischer Scientific). When the temperature reached 40°C, the tubes were centrifuged (MSE Mistral 1000) for 8 min at 1200 m/s2 and for 5 min at 600 m/s2 to obtain fully skimmed (0·0 % fat) and semi-skimmed (1·6 ± 0·2 % fat) milk, respectively. The fat layer was then carefully removed with a plastic spoon, the samples were equilibrated to room temperature, and the fat concentration in milk was measured. If the desired fat concentration was not reached, the number of rotations was adapted and centrifugation repeated until satisfactory results were achieved.
Analytical methods
Milk, whey, yogurt and cheese
Iodine concentrations were measured after alkaline extraction with tetramethylammonium hydroxide (TMAH) (Tama pure-AA TMAH 25 %; Tama) by multicollector inductively coupled plasma mass spectrometry (ICP-MS) (Neptune, Thermo Finnigan) using isotope dilution analysis with 129I (Standard Reference Material 4949C; National Institute of Standards and Technology) and a tellurium standard (AppliChem) for mass bias correction according to the isotope ratio method as previously described(Reference Dold, Baumgartner and Zeder38). The following modifications were applied to the analysis of cheese samples: we defrosted samples overnight, homogenised fresh cheese samples using a homogeniser (Polytron PT 1200 E; Kinematica), finely grated semi-hard cheese samples, weighted 0·5 g of sample, extracted samples using 1·25 ml TMAH (25 %), transferred 4 ml and added 6 ml ultrapure water (>18·2 MΩ × cm) to reach a final volume of 10 ml. Duplicate analysis was performed on 20 % of milk, whey and yogurt samples, and on 27 % of cheese samples. Certified whole-milk powder (Standard Reference Material 1549a; National Institute of Standards and Technology) was used as control material with each run of analysis, and measured values (3244 ± 130 ng iodine/g; n 62) were within the certified range as specified by the manufacturer. Inter-assay precision was 3·7 % and intra-assay CV was 1·9 %.
Milk samples from the heat treatment part of experiment 3 were analysed for iodine speciation at the Swiss Federal Food Safety and Veterinary Office using ion chromatography (Dionex ICS 5000+ system; Thermo Scientific) coupled with ICP-MS (iCAP Q; Thermo Scientific) without any sample preparation other than 23- to 25-fold dilution with ultrapure water, followed by filtration through a 0·45-μm syringe filter. Different iodine-containing species were separated on a Dionex IonPac AS 19 column with a pre-column AG19. The resulting 127I signals were monitored on ICP-MS. Standard solutions of KI and potassium iodate (Merck Chemicals) were diluted freshly to prepare calibration curves in the range between 0 and 16 μg/l iodine.
All milk samples from experiment 1 and four milk samples collected from bulk tanks per 2-week period from experiment 2 were freshly analysed by control authorities for fat, protein, lactose and casein using Fourier-transformed IR spectrophotometry (Freiburgisches Agro-Lebensmittellabor, Posieux) and a standardised method (ISO 9622:2013; Assessorato Agricoltura e Risorse Naturali, Aosta). Fat concentrations in milk samples from experiment 3 were analysed using an ultrasonic milk analyser (Lactoscan SAP, Milkotronic Ltd).
Feed
Feed samples from experiment 1 were analysed for DM, crude protein, acid- and neutral-detergent fibre, ash, Ca, P, Mg, K, Na, Cu, Fe, Mn, Zn and Se, all as recently described(Reference Schlegel, Wyss and Arrigo39), and for iodine and thiocyanate. In forage and MR samples from experiments 1 and 2, iodine was determined at the Swiss Federal Food Safety and Veterinary Office by ICP-MS (Thermo Element-XR; Thermo Fisher Scientific) after microwave-induced combustion with a modified procedure from Mesko et al. (Reference Mesko, Mello and Bizzi40) as previously described(Reference van der Reijden, Galetti and Hulmann8) in single measurements and using a certified hay powder (Standard Reference Material BCR-129; Institute for Reference Materials and Measurement) for quality control. In concentrates from experiment 1 and in previously freeze-dried barley patties from experiment 2, iodine was determined after alkaline extraction with TMAH by ICP-MS as previously described(Reference Dold, Baumgartner and Zeder38), with the following modifications. We diluted 250 mg of crushed sample using a mortar in 5 ml ultrapure water prior to TMAH addition; we further diluted barley patties-extracted samples (except dose 0) ten times before analysis. We prepared and measured all samples in triplicates. We monitored the accuracy of the analyses using a laboratory feed control sample in each run of analysis, both at its native iodine concentration and spiked with 4000 ng iodine/g (amount recovered was 99 ± 0·6 %). Thiocyanate concentration was determined in single analysis by pyridine barbituric acid method(Reference Asmus and Garschagen41, Reference Below and Weuffen42) at Thüringer Landesanstalt für Landwirtschaft, Jena, Germany, using a laboratory internal control (rapeseed meal; 154·6 mg thiocyanate/kg) (Agrokorn AG) for quality control.
Water
Iodine concentration was measured using the Pino modification(Reference Pino, Fang and Braverman43) of the Sandell–Kolthoff reaction. We used laboratory-specific control samples (70 ± 3 and 190 ± 5 μg iodine/l) for quality control.
Blood plasma
Thyroid-stimulating hormone (TSH) and T4 concentrations were determined at the Clinical Laboratory of University of Zurich (Vetsuisse) on Immulite 2000 XPi (Siemens Healthcare Diagnostics GmbH) using a canine T4 chemiluminescence assay (Immulite 2000 Canine Total T4) and a canine TSH chemiluminescence assay (Immulite 2000 Canine TSH). No reference ranges specific to cows’ thyroid hormones were available. Two levels of quality control material were assayed using manufacturer control limits to monitor day-to-day assay performance.
Data analysis
Data were analysed using the R statistical programming environment (version 3.3.1; R Core Team 2016, R Foundation for Statistical Computing) with package nlme (Reference Pinheiro, Bates and DebRoy44).
In experiment 1, we assessed data normality using the Shapiro–Wilk W-test. When departing significantly from normality (W < 0·97), we applied logarithmic and square root transformations, and chose the transformation with P > 0·05. If none of the transformations was satisfactory, we used the untransformed data for the analysis and carefully evaluated the models’ residuals using the Tukey–Anscombe plot. We computed the variable ‘feed iodine concentration’ by adding iodine from the basal diet – as calculated from the MR and concentrates and their individual consumption – to supplemental iodine and by correcting for individual DM consumption. We investigated the effects of time (experimental day), treatment (feed iodine concentration (mg iodine/kg of DM), as a continuous variable) and of their interaction (as fixed factors), and the effect of animal (as a random factor) on MIC (μg/l), total milk iodine (mg/d), total iodine intake (mg/d), feed-to-milk iodine transfer (%), milk yield (kg/d), fat (g/100 g), protein (g/100 g), lactose (g/100 g), casein (g/100 g), TSH (ng/ml) and T4 (μg/dl) using mixed effect model (MEM) analysis. When the assumption of homoscedasticity was breached (i.e. variance of the dependent variable increased with increasing feed iodine concentration), we weighed for treatment categories (i.e. supplementation levels 0, 0·5, 0·7, 1 and 2 mg iodine/kg of DM) to control for heterogeneity of variance. When predictors in the MEM exceeded moderate collinearity (>0·4), we replaced the continuous treatment variable (feed iodine concentration) with the categorical treatment variable (supplementation levels). For MIC only, using SPSS (IBM SPSS Statistics, version 23.0) curve estimations, we derived the equation that describes MIC saturation curve over time and we provided a model for the prediction of MIC as a function of supplementation level and experimental day. On day 21, we evaluated the dose–response relationship between feed iodine concentration and each dependent variable (MIC, total milk iodine, total iodine intake, feed-to-milk iodine transfer, milk yield, TSH and T4) by computing an adjusted R 2 from regression analysis. For MIC only, we provided a model for the prediction of MIC as a function of feed iodine concentration. On day 21, we investigated the effect of time-of-day (morning v. evening, as a fixed factor) and of animal (as a random factor) on MIC using MEM analysis. Additionally, we calculated the feed-to-milk iodine transfer rate using individual feed intake, feed iodine concentration, milk yield and MIC on days 3, 7, 14 and 21 using data averaged over the previous 3, 4, 7 and 7 d, respectively, and investigated whether milk yield (kg/d) affected feed-to-milk iodine transfer rate by MEM analysis. Finally, we tested if fat and protein (as fixed factors) and animal (as a random factor) affected MIC by MEM analysis.
In experiment 2, we calculated median and interquartile range (IQR) iodine concentration (μg/kg) for each type of product (Valle d’Aosta Fontina PDO, Toma, Reblec, Tomino, yogurt) by stage of production (milk, whey, curds after whey drainage, 24 h after pressing in mould and during cellar-ripening at 14, 28 and 56 d (Toma) and at 14, 42 and 84 d (Valle d’Aosta Fontina PDO)) and by iodine supplementation level. We evaluated milk iodine partitioning into whey and iodine recovery in the end-product by applying the following factory-specific historic yielding factors: 10 % for Valle d’Aosta Fontina PDO, 9 % for Toma, 22 % for Reblec, 19 % for Tomino and 100 % for yogurt. We evaluated the dose–response relationship by fitting linear regression models of MIC and iodine concentration in the resulting end-product. We evaluated the change in iodine concentration of semi-hard cheeses during ripening by fitting linear regression models of iodine concentration in cores collected 24 h after pressing in mould, and during cellar-ripening at 14, 28 and 56 d (Toma) and at 14, 42 and 84 d (Valle d’Aosta Fontina PDO). Using our iodine data from all five types of production and milk, we provided a linear model for the prediction of iodine concentration in semi-hard cheese, fresh cheese and yogurt as ready for consumption as a function of milk iodine.
In experiment 3, we investigated the effect of skimming (as a fixed factor) and farm (as a random factor) on MIC using MEM analysis. We investigated the effect of heat treatment (as a fixed factor) and iodine level (as a random factor) on iodide and total iodine concentrations in milk by MEM analysis.
The predictors in MEMs are presented with a standardised coefficient (β) and P value. Linear regression fits are presented as adjusted R 2 and P value. Significance level was set to <0·05.
The contribution of dairy products to iodine RDA, according to varying iodine concentrations in cow feed, was estimated with regression equations from experiments 1 and 2. The resulting iodine concentration was combined with adult daily consumption data of dairy products from a recent Swiss nationwide survey(45). The applied mean (2·5 % CI, 97·5 % CI) consumptions were: 110 (100, 120) g/d milk, 26·7 (24·2, 29·3) g/d semi-hard cheese, 23·6 (21·6, 25·7) g/d fresh cheese (also includes soft cheese, semi-soft cheese and spreadable cheese) and 52·6 (48·8, 56·5) g/d yogurt.
Results
Experiment 1: iodine transfer from feed to cows’ milk
Based on individual MR and concentrate intake recordings and their iodine concentrations (Table 1), the calculated feed iodine concentrations (mean ± sd) of the five experimental diets were 0·09 ± 0·00, 0·53 ± 0·02, 0·73 ± 0·01, 1·01 ± 0·03 and 1·92 ± 0·04 mg/kg of DM, respectively. The effects of treatment (feed iodine concentration or supplementation level, as appropriate), experimental day, and their interaction on MIC (μg/l), total milk iodine (mg/d), total iodine intake (mg/d), feed-to-milk iodine transfer (%), milk yield (kg/d), TSH (ng/ml) and T4 (μg/dl) are shown in Table 2, together with the dose–response relationship between feed iodine concentration and each variable on day 21. MIC increased over time at the supplementation levels of 0·5, 0·7, 1 and 2 mg iodine/kg of DM (all P<0·001), but not at 0 mg iodine/kg of DM (P = 0·50) (Fig. 1). After 21 d of treatment, MIC appeared to reach a near-steady state. The change in MIC over time is described as a function of supplementation level and experimental day (Equation 1):

Table 2. Milk iodine concentration (MIC), total milk iodine, total iodine intake, iodine transfer rates, milk yield and circulating thyroid hormones by experimental day and by supplementation level (n 5 per supplementation level)
(Medians and interquartile ranges (IQR))
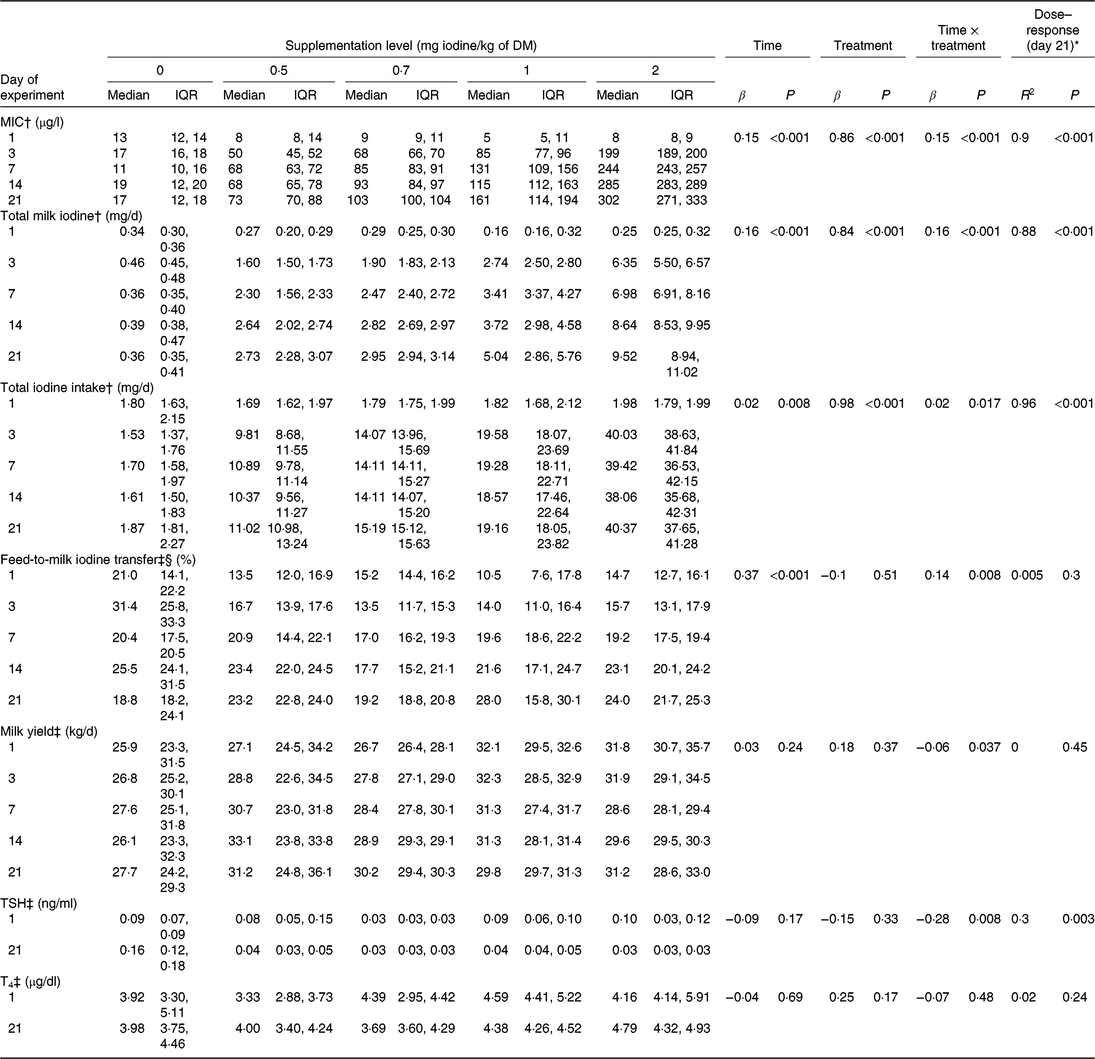
TSH, thyroid-stimulating hormone; T4, thyroxine; MEM, mixed effect model.
* Regression analysis for the evaluation of the dose–response relationship at day 21 between feed iodine concentration and dependent variables.
† Data were analysed using MEM to test the effects of time (experimental day), treatment (feed iodine concentration as a continuous variable) and their interaction (as fixed factors) and of animal (as a random factor).
‡ Data were analysed using MEM to test the effects of time (experimental day), treatment (supplementation level as a categorical variable) and their interaction (as fixed factors) and of animal (as a random factor).
§ Log-transformed data were used for MEM and regression analyses.

Fig. 1. Changes in milk iodine concentration (MIC) over time (experimental day) by treatment (supplementation level). To show the sole effect of supplementation on MIC, the values are corrected by median MIC (n 5) from the 0 mg iodine/kg of DM treatment group at each time-point, and resulted in median feed iodine concentrations from supplementation of 0·4, 0·6, 0·9 and 1·8 mg iodine/kg of DM, respectively. MIC increased over time at supplementation levels of 0·5 mg (□), 0·7 mg (○), 1 mg (Δ) and 2 mg (+) iodine/kg of DM (all P < 0·001).
There was a strong linear relationship between feed iodine concentration and MIC on d 3 (R 2 0·89; P < 0·001), 7 (R 2 0·91; P < 0·001), 14 (R 2 0·92; P < 0·001) and 21 (R 2 0·90; P < 0·001). Based on the results of day 21, MIC is described as a function of feed iodine concentration (Equation 2):

MIC was lower in morning milk (median (IQR): 94 (66, 186) μg/l) than in evening milk (114 (75, 204) μg/l) (β −0·10; P < 0·001), and milk yield in the morning (16·0 (14·8, 19·1) kg) was greater compared with the evening (13·9 (12·4, 14·3) kg) (β 0·91; P < 0·001). Nevertheless, milk yield did not correlate with feed-to-milk iodine transfer (β 0·06; P = 0·65).
Milk had a median (IQR) concentration (g/100 g) of fat, protein, lactose and casein of 4·2 (3·8, 4·5), 3·1 (2·9, 3·3), 4·8 (4·8, 4·9) and 2·4 (2·3, 2·6), respectively. Milk protein, lactose and casein concentrations increased with time (β 0·04, P < 0·001; β 0·02, P = 0·030; and β 0·04, P < 0·001, respectively) but not fat (β −0·01, P = 0·34). All were independent of feed iodine concentration (all P > 0·32) and were not affected by the time × treatment interaction (all P > 0·10). Finally, milk fat and protein concentration did not affect MIC (β −0·01, P = 0·32; β 0·01, P = 0·44, respectively).
Experiment 2: iodine transfer from milk to dairy products
Iodine concentrations found in the dairy products (Valle d’Aosta Fontina PDO, Toma, Reblec, Tomino, yogurt) are presented as median (IQR), by stage of production (milk, whey, curds after whey drainage, 24 h after mould pressing and after cellar-ripening for 14, 28 and 56 d (Toma) and for 14, 42 and 84 d (Valle d’Aosta Fontina PDO)) and by supplementation level (Table 3). There was a strong linear association between MIC and iodine concentration in the end-product for Valle d’Aosta Fontina PDO (R 2 0·91, P<0·001), Toma (R 2 0·98, P < 0·001), Reblec (R 2 1·00, P < 0·001), Tomino (R 2 1·00, P < 0·001) and yogurt (R 2 1·00, P < 0·001). Median (IQR) iodine partitioning from milk into whey was 75 (72, 78) % for Valle d’Aosta Fontina PDO (n 12), 84 (83, 87) % for Toma (n 12), 79 (78, 80) % for Reblec (n 6), 82 (82, 82) % for Tomino (n 3) and –4 (–7, –3) % for yogurt (n 3). Consequently, median (IQR) iodine recovery in the cheeses and the yogurt were 25 (22, 28) % (n 12), 16 (13, 17) % (n 12), 21 (20, 22) % (n 6), 18 (18, 18) % (n 3) and 104 (103, 107) % (n 3), respectively. These summary data do not include data from the supplementation period at dose 0 mg iodine/kg of DM due to the high variability of percentage data for values close to 0. Ripening had no effect on iodine concentration in cores collected 24 h after mould pressing, and cellar-ripening for 14, 28 and 56 d (Toma) and for 14, 42 and 84 d (Valle d’Aosta Fontina PDO) for any supplementation levels (all R 2 ≤ 0·1, P>0·05). Based on our results, iodine concentration in semi-hard cheese, fresh cheese and yogurt can be calculated as a function of MIC using the following equations:



Table 3. Iodine concentrations in all types of products (Valle d’Aosta Fontina Protected Designation of Origin (PDO), Toma, Reblec, Tomino, yogurt) by the stage of production (milk, whey, curds after whey drainage, 24 h after mould pressing, at 14, 28, 56 d of ripening (Toma) and at 14, 42, 84 d of ripening (Valle d’Aosta Fontina PDO)) at the four supplementation levels
(Medians and interquartile ranges (IQR); single values)

* At a theoretical DM intake of 14·5 kg/d.
The milk compositional analysis (n 16) showed a median (IQR) concentration (g/100 g) of fat, protein, lactose and casein of 3·3 (3·2, 3·4), 3·3 (3·2, 3·3), 4·9 (4·8, 4·9) and 2·5 (2·5, 2·5), respectively.
Experiment 3: milk heat treatment and skimming
Iodine speciation in high- and low-iodine milks showed iodide as the only detectable iodine fraction in both raw and heat-treated milk. In high-iodine milk, we measured a mean ± sd iodide concentration of 949 ± 74 and 980 ± 25 μg/l and an iodine concentration of 1062 ± 3 and 1052 ± 19 μg/l in samples prior to and post-treatment, respectively. This resulted in an iodide–iodine ratio of 89·4 and 93·2 %, respectively. In low-iodine milk, we measured an iodide concentration of 57 ± 1 and 64 ± 3 μg/l and an iodine concentration of 80 ± 0 and 80 ± 1 μg/l in samples prior to and post-treatment, respectively. This resulted in an iodide–iodine ratio of 71·2 and 80·5 %, respectively. Heat treatment had no effect on milk iodide fraction (β 0·04, P = 0·48, n 4) or on total iodine (β 0·01, P = 0·46, n 4).
Median (IQR) MIC was 88 (58, 104) μg/l for the full fat milk (4·3 (4·2, 4·4) % fat; n 18); 90 (86, 135) μg/l for the semi-skimmed milk (1·7 (1·6, 1·8) % fat; n 34); and 89 (65, 122) μg/l for the fully skimmed milk (0·0 % fat; n 19). Skimming had a slight but significant effect on MIC (β –0·02, P < 0·001).
Calculation of contribution of milk and dairy products to iodine supply
The mean (95 % CI) contribution of dairy products to iodine supply in Swiss adults’ RDA was estimated at 13 (12, 14) %, 26 (24, 28) % and 52 (48, 56) % with a supplementation level in the dairy diet of 0·5, 1 and 2 mg iodine/kg of DM, respectively (Table 4). A 100 % coverage of adults’ RDA would be achieved with 3·8 mg iodine/kg of DM, assuming validity of the regression up to that supplementation level.
Table 4. Contribution of milk and dairy products to the adults’ RDA for iodine according to Swiss dairy consumption data*
(Mean values and 2·5 % confidence intervals, 97·5 % confidence intervals)

* Feed-to-milk iodine model from experiment 1 (Equation 2) and milk-to-dairy iodine model from experiment 2 (Equations 3, 4, and 5). Mean (2·5 % CI, 97·5 % CI) adult dairy consumption (n 2085) in Switzerland is 110 (100, 120) g/d for milk, 26·7 (24·2, 29·3) g/d for semi-hard cheese, 23·6 (21·6, 25·7) g/d for soft cheese (includes semi-soft cheese, fresh cheese and spreadable cheese) and 52·6 (48·8, 56·5) g/d for yogurt(45).
† Fresh cheese iodine concentration (μg/kg) was used to calculate the iodine intake from soft cheese (including semi-soft cheese, fresh cheese and spreadable cheese).
Discussion
Relationship of iodine in feed with cows’ milk
We found a strong linear relationship between feed iodine concentration and MIC in experiment 1 (R 2 0·90, P < 0·001) after 21 d of iodine supplementation. This finding is in agreement with the results of Franke et al. (Reference Franke, Meyer and Wagner18), who also showed a strong linear relationship (R 2 > 0·94) between feed and milk iodine concentrations regardless of the supplementation source and regardless of the dairy diet type, even though these may differ in the presence or absence of iodine antagonists. Moschini et al. (Reference Moschini, Battaglia and Beone22) (R 2 0·90, P < 0·001) and Borucki Castro et al. (Reference Borucki Castro, Berthiaume and Robichaud25) (R 2 0·8, P < 0·001) also demonstrated a strong linear dose–response relationship between feed iodine concentration and milk iodine. In contrast, Norouzian(Reference Norouzian31) suggested a logarithmic dose–response relationship, due to, these authors explained, the mammary gland acting as a bioregulator for iodine excretion leading to a reduction in secretion ratios at higher levels of supplemental iodine. However, this was shown at higher supplementation doses. When MIC was plotted against daily supplemental iodine fed to each cow (i.e. 0, 12·5, 17·0 and 36·5 mg iodine/d in experiment 1 and 0, 10·2, 14·5 and 28·7 mg iodine/d in experiment 2), the two slopes were highly compatible (MIC (μg/l) = 7·4 × supplemental iodine (mg/d) – 13·6; and 6·9 × supplemental iodine (mg/d) – 14·2 for experiments 1 and 2, respectively). The slopes of linear relationships between feed iodine concentration or daily supplemented iodine and MIC were lower than the ones available from other studies(Reference Schone, Leiterer and Lebzien17–Reference Borucki Castro, Berthiaume and Robichaud25). Not only iodine intake from the diet but also other dietary factors potentially affect MIC and might explain these differences(Reference Flachowsky, Franke and Meyer9). MIC is known to decrease with increasing concentrations of iodine antagonists in the cows’ diet, such as thiocyanates and isothiocyanates found in all Brassica-based feeds (e.g. rapeseed)(Reference Franke, Meyer and Wagner18, Reference Laurberg, Andersen and Knudsen46–Reference Gaitan48). However, thiocyanate concentrations analysed in the diet components in experiments 1 and 2 were low (<7·1 v. 154·6 mg/kg found in rapeseed meal used as internal control). Nitrate, which can occur in substantial amounts depending on the season (high in autumn), farm management and plant stress conditions, is also an iodine antagonist that may reduce MIC(Reference Rasby, Anderson and Kononoff49, Reference Mervish, Pajak and Teitelbaum50). In both experiments, the diet was composed of >80 % grass-based feeds on a DM basis with potentially elevated nitrate concentrations, compared to the eight experiments considered by Flachowsky et al. (<25 % herbage on DM basis)(Reference Flachowsky, Franke and Meyer9, Reference Schone, Leiterer and Lebzien17–Reference Borucki Castro, Berthiaume and Robichaud25). Thus, nitrate concentration of the cows’ diet might partly explain the difference in MIC at similar supplementary dietary iodine levels. However, dietary nitrate concentration was not reported in any of the dose–response studies described in literature, and it was not determined in our two studies either, leaving this question unanswered. Other factors that might influence MIC and thus limit our analysis of external consistency are cattle breed and milk yield, for which information is scarce and results are controversial(Reference Flachowsky, Franke and Meyer9, Reference Battaglia, Moschini and Giuberti20, Reference Moschini, Battaglia and Beone22). In experiment 1, we used cows of the high-performing breed, Holstein-Friesian, widely used in iodine studies, whereas in experiment 2, we investigated cows of the low-yielding dual-purpose breed, Aosta Red Pied. Mean milk yield was 29·3 kg/d in experiment 1 and 17·2 kg/d in experiment 2. The feed-to-milk iodine transfer rate in experiment 1 was 18·8–28·0 %, which is in the range of iodine transfer rates (15–26 %) found in two other experiments with similar milk yields (31·7 ± 1·8 and 32·1 ± 4·9 kg/d), supplementation doses (1·55 and 1·71 mg iodine/kg of DM) and MIC (227 and 240 μg/l)(Reference Moschini, Battaglia and Beone22). However, in other studies, higher transfer rates of 30–56 % were found(Reference Schone, Leiterer and Lebzien17, Reference Franke, Meyer and Wagner18).
Effects of milk processing on iodine concentration in dairy products
We found a strong linear association between MIC and dairy products (all R 2 > 0·90, all P<0·001). We found that a large proportion of iodine is lost in whey (≥75 %) during production; thus, iodine recovery in cheese was ≤25 %. Despite these low recovery rates, iodine concentrations in the final products increased to levels relevant for human nutrition due to concomitant moisture loss during cheese production (about 80 % for fresh cheeses and 90 % for semi-hard cheeses). Following curdling and ripening of the two semi-hard cheeses (Valle d’Aosta Fontina PDO and Toma), iodine concentration in the cheese increased to about 1·6–2·3 times (after curdling) and 1·6–2·6 times (after ripening) that of milk. This is in agreement with the results of Moschini et al. (Reference Moschini, Battaglia and Beone22) for Grana Padano PDO (a hard cheese), where they reported a 1·7-fold increase in iodine concentration in the curds compared to that of the milk. Iodine concentrations of dairy products in our study were in the magnitude of cheese iodine concentrations reported by surveys in Switzerland (median iodine concentration of 93–301 μg/kg in hard and semi-hard cheeses, 83–101 μg/kg in fresh and soft cheeses and 156–169 μg/kg in yogurt)(Reference Haldimann, Alt and Blanc2, 3). However, we specifically used iodine-free salt during cheese production, whereas for the cited values, this information is unknown because the analysed cheeses had been purchased from retail outlets, and iodised salt was not declared on the labels at that time. The adventitious iodine contamination during the production of Tomino cheese at a supplementation level of 0 mg iodine/kg of DM diet is indicative of the potential effect of iodine addition via iodised salt during production (2 μg/l in milk v. 146 μg/kg in the curds of contaminated Tomino cheese, whereas the expected value would have been 1·9 μg/kg in the curds based on Equation 4. These data suggest that iodine contribution from iodised salt used during cheese production obviates the efforts to increase dairy iodine concentration via the modulation of feed iodine. The use of iodised salt at nutritionally relevant amounts could be an easily applicable way to increase iodine concentration in cheese in a controlled manner. However, non-iodised salt is still used in the production of cheese in Switzerland, driven by the Swiss declaration duty, which stipulates that cheese produced using iodised salt, but not non-iodised salt, must have an ingredient label(3, 35).
We found that MIC was consistently higher in semi-skimmed and fully skimmed than in full-fat milk; the magnitude of difference was, however, negligible. Three studies that investigated MIC in commercial retail milks with different fat concentrations (whole, semi-skimmed and fully skimmed milks) with multiple samplings over several months or years found differences in MIC related to skimming(Reference Arrizabalaga, Jalón and Espada6, Reference Jahreis, Leiterer and Fechner33, Reference Rasmussen, Carlé and Jørgensen34). In contrast, Soriguer et al. (Reference Soriguer, Gutierrez-Repiso and Gonzalez-Romero32) described higher iodine concentrations in commercial retail skimmed milks compared with semi-skimmed and whole milks. Since their study analysed retail milk samples collected at a single time-point, however, spaced out over 10 months, it might be that other factors such as feeding, days in milk, etc., might have acted as confounders on iodine in different skimmed milks.
Our results suggest that heat treatment does not affect iodine concentration. This is in agreement with some studies(Reference Wheeler, Fleet and Ashley28, Reference Aumont, Lequerrec and Lamand29) but not others(Reference Pedriali, Giuliani and Margutti30, Reference Norouzian31), in which it was postulated that a heat-induced decrease in iodine is explained by the sublimation of iodide fraction(Reference Norouzian31). When we investigated the concentration of iodide fraction in milks, we found that it did not differ between pre- and post-heat treatment. The resulting iodide–iodine ratio was of 71–89 % in raw milks and 81–93 % in pasteurised and homogenised milks. This was somewhat lower compared with previous speciation studies, which found about 80–95 % of inorganic iodine (predominantly in the form of iodide)(Reference Norouzian31, Reference Leiterer, Truckenbrodt and Franke51, Reference Flynn and Cashman52) but similar ratios have also been reported(Reference Leiterer, Truckenbrodt and Franke51).
Contribution of milk-derived iodine to dietary need of the population
Considering the current contribution of iodised salt to the total dietary iodine intake (54 %)(Reference Haldimann, Bochud and Burnier53) and the most recent results on the consumption of milk and dairy products in Switzerland(45), the desirable target MIC should be set to 150–300 μg/l (achievable with a dairy cows’ iodine supplementation of 1–2 mg iodine/kg of diet DM). This would lead to a contribution of about 25–50 % to the RDA of adults from milk and dairy products. This calculation does not consider the potential increment of iodine intake if iodised salt was used during cheese production. The target MIC could be set even higher in light of the WHO-advocated reduction in salt consumption as a measure to reduce the risk of cardiovascular diseases(54), which was adopted by the Swiss authorities(55). Examples from other countries show that milk and dairy products have the potential to substantially contribute to the RDA if intakes and MIC are within a relevant range. For example, in the UK, milk and dairy products contribute 49–73 % to RDA (306–458 μg/l, 239 g/d)(Reference Payling, Juniper and Drake56, 57), and 91–115 % is covered in the USA (365–464 μg/l, 373 g/d)(Reference Pearce, Pino and He58–60). Recent studies, however, indicated that MIC in Switzerland is lower (55–71 μg/l in organic milk, 93–111 μg/l in conventional milk)(Reference van der Reijden, Galetti and Hulmann8, Reference Walther, Wechsler and Schlegel10), covering only about 9–19 % of the RDA. Lastly, the recent trend towards a gradual decrease in milk consumption in high-income countries, partially compensated by a slight increase in cheese consumption, should be anticipated when setting the target MIC(Reference Kearney61).
Strengths and limitations of the study
To our knowledge, we presented the first dose–response study in high-yielding dairy cows fed mainly with grassland-based diets, diets which are typical for various regions within and outside of Europe. Moreover, experiment 1 was conducted under highly controlled conditions in an experimental barn, where individual feed intakes were recorded daily and iodine supplementation was individually adapted on a weekly basis to reach the predefined target supplementation levels. As far as we know, experiment 2 was the first study that systematically investigated iodine losses during milk processing on five different dairy products and monitored the effect of cellar-ripening for up to 3 months. Moreover, we measured all samples by ICP-MS, a sensitive and accurate method to determine iodine in complex sample matrices such as milk and cheese. Limitations included that, in experiments 1 and 2, we only assessed feed thiocyanate concentrations and did not measure other potential dietary antagonists of iodine such as nitrate. Nevertheless, it would have been difficult to interpret the effect of such antagonists because no other dose–response study considered components other than glucosinolates. Moreover, in experiment 2 we only considered iodine losses during milk processing and did not investigate the effect of the usage of iodised salt on iodine concentrations of the cheeses.
Conclusions
Our findings showed a strong linear relationship in iodine concentrations between dairy feed and milk and between milk and dairy products at supplementation levels between 0·5 and 2 mg iodine/kg of dairy cows' diet DM based on feeds from grasslands. These provided a quantification of the extent to which iodine concentration in milk and dairy products can be varied through supplemental iodine in cow feed. This opens the possibility to establish milk as a relevant and reliable source of dietary iodine. The desirable target MIC is 150–300 μg/l, achievable with an iodine supplementation of 1–2 mg iodine/kg of dairy cows diet DM, leading to a contribution of about 25–50 % to the RDA of adults. Nevertheless, two considerations must be made when adjusting cattle diet formulations. (1) Many factors (including feed iodine concentration, iodine antagonists in feed, cattle breed, milk yield, teat disinfection, season, farm management) can potentially blur the dose–response relationship between feed iodine concentration and milk iodine; thus feeding recommendations have to be formulated considering all the available data. (2) The dose–response relationship between MIC and iodine concentration in dairy products is likely applicable to similar dairy products, but must consider if iodised salt is used during processing, as this could greatly increase iodine concentrations in cheeses.
Acknowledgements
We thank Lukas Eggerschwiler, Jean-Luc Bonny, Walter Glauser and Andreas Gutzwiller for their assistance during the experiment held at Agroscope in Posieux, Switzerland. We thank Luca Vernetti-Prot, Germano Turille, Michela Cabraz, Michel Oreiller, Rudy Guichardaz, Rudy Grange, Angelo Papa, Luca Prisant and Madeline Koczura for their assistance during the experiment held at Institut Agricole Régional in Aosta, Italy. We are grateful to Sébastien Dubois and his team, Rafael Aubert, Andrea Mannhart, Sarah Bregant, Carmen Häller and Barbara Riond for assistance with laboratory analyses.
This work was supported by the Swiss Federal Food Safety and Veterinary Office (M. B. Z.; grant no. 2014-11-06/21) and the Coop Research Program by the World Food System Centre (I. H.-A.; grant no. 2015-10).
I. H.-A., M. B. Z., M. H., P. S., J. B. and M. K. formulated the research questions and designed the studies. O. L. V., V. G., C. Z., A. B., J. B. and P. S. carried out the experiments and collected the samples. O. L. V., V. G., M. H., C. Z. and A. K. were responsible for laboratory analyses; O. L. V. and V. G. performed statistical analysis and wrote the manuscript with support from I. H.-A., M. B. Z., M. H., M. K., J. B. and P. S.
The authors declare that there are no conflicts of interest.