Elucidation of the risk factors for cognitive decline is required to prevent and possibly reverse age-related cognitive impairment in elderly people. In this respect, the one-carbon metabolism is of interest because plasma concentrations of homocysteine and related B-vitamins have been associated with cognitive impairmentReference Selhub, Bagley, Miller and Rosenberg1–Reference Malouf and Grimley Evans6. Moreover, choline plays an important role in normal brain development, in particular for memory and learningReference Zeisel7.
The metabolites of the one-carbon metabolism are closely interrelatedReference Holm, Ueland and Vollset8, Reference Melse-Boonstra, Holm, Ueland, Olthof, Clarke and Verhoef9. Homocysteine is located at a critical metabolic branch point with ramification to methyl- and sulphur group metabolism (Fig. 1). Homocysteine is formed from the essential amino acid methionine. Methionine is activated by its conversion to S-adenosylmethionine, and S-adenosylmethionine is required for methylation of many acceptor substrates, such as DNA, RNA, lipids, proteins, phosphatidylethanolamine, creatine, myelin basic protein and neurotransmittersReference Miller10. Methylation of, for example, phosphatidylethanolamine to form phosphatidylcholine, is important to maintain myelin sheaths of nerve tissue and thereby for central nervous system structure and functionReference Zeisel7. Homocysteine is remethylated to methionine by the cobalamin (vitamin B12)-dependent enzyme, methionine synthase, which uses 5-methyltetrahydrofolate as a methyl donor. Deficiencies of folate and cobalamin impair this conversion and result in increased homocysteine concentrationsReference Stabler and Banjeree11. In a few tissues, predominantly the liver and kidneys, homocysteine remethylation is also catalysed by the enzyme betaine-homocysteine methyltransferase. Methionine and dimethylglycine (DMG) are the products of this reaction. The methyl donor, betaine, is formed from choline, which also is a precursor for the neurotransmitter acetylcholineReference Ueland, Holm and Hustad12. Conceivably, impaired one-carbon metabolism may be associated with cognitive impairment by affecting neurotransmitter metabolism and central nervous system function.
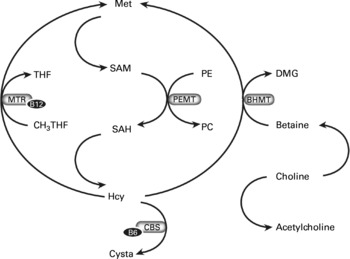
Fig. 1 B-vitamins (B6 and B12), homocysteine (Hcy), choline, betaine and the one-carbon metabolism. The conversion from Hcy to methionine (Met) can be catalysed by methionine synthase (MS) and betaine-homocysteine methyltransferase (BHMT). The conversion via MS requires cobalamin and 5-methyltetrahydrofolate (THF) as a methyl donor and couples folate metabolism to the choline-betaine pathway. Choline is provided by food or can be formed de novo via sequential S-adenosylmethionine (SAM)-dependent methylation of phosphatidylethanolamine (PE) to form phosphatidylcholine (PC) by phosphatidylethanolamine N-methyltransferase (PEMT). Choline is a precursor for the neurotransmitter acetylcholine or it is oxidized to betaine. Betaine donates its methyl group directly to homocysteine for the conversion into methionine, and results in dimethylglycine (DMG) in the BHMT reaction. CBS, cystathionine-β-synthase; CH3THF, methyltetrahydrofolate; cysta, cystathionine; MTR, methionine synthase; SAH, S-adenosylhomocysteine.
Following the epidemiological associations found between homocysteine, cobalamin and folate with cognitive performanceReference Selhub, Bagley, Miller and Rosenberg1–Reference Malouf and Grimley Evans6, recent intervention trials investigated the efficacy of homocysteine lowering with B-vitamin supplementation on cognitive function in elderly peopleReference McMahon, Green, Skeaff, Knight, Mann and Williams13–Reference Durga, van Boxtel and Schouten15. However, the associations of plasma concentrations of choline, betaine, DMG and methionine with cognitive performance have not been explored yet. A recently conducted efficacy trial, investigating the efficacy of oral cobalamin with or without folic acid supplementation on cognitive functionReference Eussen, de Groot and Joosten14, provided such an opportunity. In addition, we assessed whether supplementation with cobalamin and folic acid altered plasma concentrations of choline, betaine and DMG, and consequently, whether alterations in these metabolites were associated with improvements in cognitive function.
Subjects and methods
Participants
Elderly men and women aged 70 years or older were screened for participation in a randomized double-blind placebo-controlled trial that studied the efficacy of oral cobalamin supplementation on cognitive performanceReference Eussen, de Groot and Joosten14. Individuals were included when they had mild cobalamin deficiency, defined as serum cobalamin concentrations between 100 and 300 pmol/l in combination with plasma methylmalonic acid concentrations ≥ 0·32 μmol/l and serum creatinine concentration ≤ 120 μmol/l to exclude severe impairment of renal functionReference Baik and Russell16. Other exclusion criteria were history of cobalamin deficiency, use of cobalamin (>50 μg/d) or folic acid (>200 μg/d) supplementation or injections, surgery or diseases of the stomach or small intestine, anaemia, life-threatening diseases, severe hearing or visual problems, and severe cognitive impairment, which was defined by a score < 19 points on the Mini-Mental State Examination (MMSE). An additional sample of individuals with adequate cobalamin status and no severe cognitive impairment (n 40) was enrolled for cross-sectional data analysis. Figure 2 presents the recruitment procedure, study design and flow of participants. The Medical Ethical Committee of Wageningen University approved the study protocol. Daily boards and client councils gave their consent for those individuals living in an institution, and written informed consent from all participants was obtained before the start of the study.
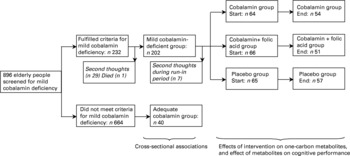
Fig. 2 Recruitment procedure, study design and flow of Dutch elderly participants.
Study design of the intervention trial
Individuals who were included in the intervention trial started with a 2-week placebo run-in period prior to randomization. Within this period, individuals were excluded from further participation if compliance (intake of capsules) was < 90 %, or if they scored < 19 points on the MMSE. Eligible participants were randomized to receive 24 weeks of treatment in a parallel group design with daily oral doses of 1000 μg cobalamin, a combination of 1000 μg cobalamin and 400 μg folic acid, or a placebo capsule. Randomization was stratified according to methylmalonic acid concentration at the screening visit (below and above 0·45 μmol/l), age (below and above 80 years), sex and MMSE (below and above 24 points).
Blood collection and biochemical analyses
A blood sample was collected at the screening and baseline visit and after 12 and 24 weeks of supplementation. A blood sample for measurement of methionine, total homocysteine (tHcy), choline, betaine and DMG was collected into a 10 ml Vacutainer® tube containing EDTA. This blood sample was placed in ice-water and centrifuged at 2600 rpm for 10 min at a temperature of 4°C within 30 min of collection. All plasma samples were stored at − 80°C prior to laboratory analyses. Plasma concentrations were determined by a method based on methylchloroformate derivatization and GC–MS (methionine and tHcy)Reference Windelberg, Arseth, Kvalheim and Ueland17 and a modification of a method based on liquid chromatography tandem MS (choline, betaine and DMG)Reference Holm, Ueland, Kvalheim and Lien18. Analytical CV of the assays for methionine, tHcy, choline, betaine and DMG were < 3·4, < 2·2, < 10, < 10 and < 10 %, respectivelyReference Windelberg, Arseth, Kvalheim and Ueland17, Reference Holm, Ueland, Kvalheim and Lien18.
Assessment of cognitive function
Six trained and registered neuropsychologists performed cognitive testing of the participants during a 1·5–2 h session during the run-in period and at week 24 of intervention. The cognitive test battery consisted of tests that have been shown sensitive to the effects of B-vitamin treatment and ageing in previous studiesReference Calvaresi and Bryan2, Reference van Asselt, Pasman and van Lier19. Individuals were screened by the MMSE, and those with an MMSE score < 19 points (maximum 30 points) were excluded because of severe cognitive impairmentReference Tombaugh and McIntyre20. Table 1 lists the order of assessment and description of the tests, including its corresponding cognitive domain and neuropsychological focus. All these single tests were clustered into domains of attention, construction, sensomotor speed, memory and executive function, as indicated in the statistical methods. Three out of five domains were assessed by at least two tests that measured different aspects and/or degrees of complexities, which has been proposed as the preferred method for cognitive testingReference Barnes, Wilson, Schneider, Bienias, Evans and Bennett21. The advantages of clustered scores are (1) the reduction of measurement errors by possible floor and ceiling effects from difficult and easy tests, respectively; (2) to be able to account for missing data; (3) data reduction and thereby reducing chance findings; and finally (4) a better sensitivity to measure cognitive changesReference Barnes, Wilson, Schneider, Bienias, Evans and Bennett21, Reference Visser22.
Table 1 Description of neuropsychological test battery with corresponding domain and neuropsychological focus
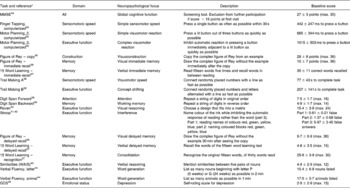
GDS, Geriatric Depression Scale; MMSE, Mini-Mental State Examination.
* Ordered by assessment.
Statistical methods
The average concentrations of the biochemical parameters at the screening and randomization visits were calculated for each individual, and defined as ‘baseline’ concentrations. Differences in concentrations of blood parameters at baseline and follow-up were assessed with a two-factor repeated measures ANOVA (two measurements × three treatment groups) that included the time × treatment interaction. Tukey post hoc tests were used to assess differences between the intervention groups.
Data on cognitive function were presented as the neuropsychological domains of attention, construction, sensomotor speed, memory and executive function. The domains of attention and construction were assessed by a single cognitive test, while the other domains were assessed by multiple tests. All crude test scores were transformed to z-scores by: z-score = ((individual result – mean result of study population at baseline)/standard deviation of study population at baseline). The multiple tests for the domains of sensomotor speed, memory and executive function were clustered to provide compound z-scores to reduce the effects of chance findings and to simplify interpretation of the cognitive data: Attention = Z Digit Span Forward; Construction = Z Rey,copy; Sensomotor speed = ( − Z Motor Planning (2)+ − Z Finger Tapping+ − Z Trail Making (part A))/3; Memory = (Z 15 Word Learning, immediate+Z 15 Word Learning, delayed+Z 15 Word Learning, recognition+Z Rey, immediate+Z Rey, delayed+Z DigitSpan Backward)/6; Executive function = ( − Z Motor Planning (3)+ − Z Trail Making (partC/partA)+ − Z Stroop (part3/part2)+Z Similarities (WAIS)+Z Raven+Z Word Fluency (Animals)+Z Word Fluency (Letter))/7.
Tests that were clustered for each cognitive domain were highly correlated (P values ranged from < 0·0001 to 0·04 for all tests). Some participants were unable to complete all tests because of performance difficulties, e.g. tiredness. Compound z-scores were calculated when data for at least two, four and five tests for the domains of sensomotor speed, memory and executive function, respectively, were available. The compound z-scores served as ‘internal’ z-scores from which z-scores at baseline and 24 weeks by study treatment were derived.
Baseline information of the present study population with mild cobalamin deficiency combined with an additional group of elderly with adequate cobalamin status enabled us to explore associations between the one-carbon metabolites and cognitive performance by means of partial correlation coefficients which were corrected for age and education.
Per protocol analyses were performed, including only the 162 participants (84 %) who completed the trial. Changes in cognitive performance associated with alterations of metabolite concentration were calculated by subtracting z-scores at the end of the intervention study by the z-scores at baseline. The potential effects of changes in metabolites on cognitive function within each domain within and across tertile categories in biochemical changes were studied by a two-factor repeated measures analyses (two measurements × three tertile categories) for each cognitive domain that included the time × tertile category interaction term. These analyses were performed with mixed models (SAS PROC MIXED procedureReference Singer and Using23), an extension from the linear regression model that includes random effects. Possible inter-investigator bias of the six neuropsychologists was entered as random effects. Statistical analyses were conducted using SAS statistical software version 9.1 (SAS Institute Inc., Cary, NC, USA).
Results
Characteristics of participants
Cross-sectional analyses have been performed for 202 elderly with mild cobalamin deficiency and forty elderly with adequate cobalamin status. The mean age of these participants was 81 (sd 6) years, 74 % of the participants were females, and 40 % of the participants lived in a care facility home. The mean score on the MMSE was 27 (sd 3) points which indicates that most of the population had intact cognitive function or only mild impairment.
The demographic, lifestyle and co-morbidity characteristics of participants are presented in Table 2. Although there were some differences with respect to lifestyle, medical history and mental status between those with mild cobalamin deficiency and those with adequate cobalamin status, there were no differences between those with mild cobalamin deficiency and the total population used for cross-sectional analyses (Table 2). Of the individuals with mild cobalamin deficiency who started with the run-in period (n 202), seven showed second thoughts, resulting in 195 participants who underwent random assignment. Two of these 195 participants dropped out during the run-in period, which left data for 193 participants who started supplementation (Fig. 2).
Table 2 Characteristics of older participants

GDS, Geriatric Depression Scale; MMSE, Mini-Mental State Examination.
* No significant differences between the three treatment groups were observed (one-way ANOVA for continuous variables and χ2 analyses for categorical variables): P >0·05.
† Values are means and standard deviations.
Values were significantly different from those of the adequate cobalamin group (unpaired t-test for continuous variables and χ2 analyses for categorical variables): ‡P < 0·05.
Blood indices and cognitive function before supplementation
Mean concentrations for methionine (25·1 μmol/l), choline (8·0 μmol/l), betaine (32·2 μmol/l) and DMG (3·7 μmol/l) in the total screened population (n 896) did not differ from those concentrations observed in the segment of participants involved in the intervention trial (n 193; P>0·05 for all indices (unpaired t-test)). Partial correlation coefficients between the blood indices of the total screened population (n 896), which were corrected for age and sex, are presented in Table 3. Consistent with the inclusion criteria of mild cobalamin deficiency, participants of the intervention trial had lower cobalamin concentrations and higher tHcy concentrations compared to the total screened population (both P < 0·0001).
Table 3 Partial Spearman rank correlation coefficients between one-carbon metabolites and vitamins with one-carbon metabolites, and between one-carbon metabolites with compound cognitive domains in a Dutch elderly population (n 242) prior to supplementation

DMG, dimethylglycine; tHcy, total homocysteine.
Partial correlation coefficients corrected for age and sex: *P < 0·05; **P < 0·0001.
Partial correlation coefficients corrected for age and education: †P < 0·10; †† < 0·05.
Partial correlation coefficients, which were corrected for age and education, revealed inverse associations of tHcy concentrations with compound scores for the domains of attention, construction, sensomotor speed and executive function. Methionine concentrations were positively associated with the domain of sensomotor speed, whereas betaine concentrations were positively associated with the domains of construction, sensomotor speed and executive function (Table 3). Further adjustment of our analysis for emotional status and other co- morbidity factors did not affect these associations (data not shown). Moreover, the observed associations did not essentially differ between mildly cobalamin-deficient participants and those cobalamin-replete subjects (data not shown).
Changes in blood indices during intervention
Table 4 presents the concentrations of vitamins and the one-carbon metabolites at baseline and at 24 weeks of supplementation. Concentrations of all metabolites in the placebo group remained stable throughout the study period. The time × treatment interaction term was significant for cobalamin, tHcy, erythrocyte folate (P < 0·0001 for these indices) and betaine (P = 0·030), which indicates differences in effects between the intervention groups. The effects of treatment did not differ between the intervention groups (no significant interaction terms) for methionine, choline and DMG. Nevertheless, concentrations of methionine and choline increased significantly by 11 and 23 %, respectively, after combined supplementation.
Table 4 Concentrations of vitamins and one-carbon metabolites at baseline and 24 weeks after supplementation with cobalamin (vitamin B12), cobalamin + folic acid or placebo in a Dutch elderly population

* Treatment effects (changes from baseline within groups) significantly different between the three treatment groups, as indicated by a significant time × treatment interaction (ANOVA): P < 0·05.
† No significant differences between the three treatment groups (ANOVA with Tukey post hoc tests): P >0·05.
‡ Significant differences between the three treatment groups (ANOVA with Tukey post hoc tests): P < 0·05.
Mean values were significantly different from those of the baseline (ANOVA repeated-measures analysis with LSMEANS): §P < 0·05.
‖ Treatment effects (changes from baseline within groups) not significantly different between the three treatment groups, as indicated by a non-significant time × treatment interaction (ANOVA): P >0·05.
Mean values were significantly different from those of the placebo (ANOVA with Tukey post hoc tests): ¶P < 0·05.
Mean values were significantly different from those of the cobalamin group (ANOVA with Tukey post hoc tests): **P < 0·05.
Cognitive performance and changes in blood indices
No differences in cognitive performance between intervention groups were observed at baseline. Since some participants were unable to complete all tests, data of 141, 158 and 151 participants were included for analyses on the domains of sensomotor speed, memory and executive function, respectively. There were no significant effects of increases in erythrocyte folate and cobalamin concentrations on cognitive performance, and this had been described elsewhereReference Eussen, de Groot and Joosten14. Table 5 presents mean changes in cognitive scores according to changes in tHcy, methionine, choline, betaine and DMG, which are categorized into tertiles. In the domain of memory, the time × tertile category interaction term was only significant for DMG (P = 0·04), and borderline significant for betaine (P = 0·07), which indicates differences in memory improvement between the tertiles. Participants with the largest increases in betaine concentrations (third tertile category; change in betaine concentrations >6·88 μmol/l) showed the highest increase in memory performance compared to those in the first and second tertiles. For DMG, participants in the second and third tertiles had a better memory performance compared to those in the first tertile. With respect to the other cognitive domains, no effects within and between tertiles were observed. Further adjustment of our analysis for emotional status and other co-morbidity factors did not affect these associations (data not shown). With respect to emotional status, there were no significant changes in Geriatric Depression Scores between the intervention groups after 24 weeks of supplementation (P = 0·32).
Table 5 Mean cognitive changes in z-scores by tertiles in changes of one-carbon metabolites due to cobalamin with or without folic acid supplementation in a Dutch elderly population (n 195)*

DMG, dimethylglycine; tHcy, total homocysteine.
a,b Mean changes in z-scores with unlike superscript letters were significantly different (P < 0·05).
* At baseline, mean compound z-scores were essentially 0 (sd 1), as a result of standardizing and combining crude cognitive scores into standardized compound z-scores. Analyses are adjusted for age, education and neuropsychologists. The cut-off points for tertile categories in changes of tHcy were − 3·95 and − 0·7 μmol/l; for methionine they were − 1·32 and 2·88 μmol/l; for choline they were − 0·17 and 1·48 μmol/l; for betaine they were − 1·43 and 6·88 μmol/l; and for DMG they were − 0·33 and 0·41 μmol/l. P values indicate tests for trend across median changes in concentrations for each tertile. Analyses are corrected for age, education and neuropsychologist.
Discussion
The present study showed that elevated plasma tHcy was associated with lower performance of attention, construction, sensomotor speed and executive function. In addition, betaine was positively associated with better performance of construction, sensomotor speed and executive function, whereas elevated concentrations of methionine were positively associated with sensomotor speed prior to supplementation. Daily oral supplementation of 1000 μg cobalamin with 400 μg folic acid for a period of 24 weeks decreased tHcy concentrations and increased betaine concentrations. There was a tendency for participants with the largest increases in betaine concentrations to show the greatest improvement in memory function.
To our knowledge, the current study is the first one that explores associations of cognitive function with choline, betaine and DMG in an elderly population. Previous animalReference Selhub, Seyoum, Pomfret and Zeisel24, Reference Kim, Miller and da Costa25 and humanReference Holm, Ueland and Vollset8, Reference Melse-Boonstra, Holm, Ueland, Olthof, Clarke and Verhoef9, Reference Jacob, Jenden, Allman-Farinelli and Swendseid26 studies indicate a strong interrelationship between the betaine-homocysteine methyltransferase and methionine synthase pathways. This is reflected in lower hepatic choline concentrations during folate deficiencyReference Kim, Miller and da Costa25, Reference Jacob, Jenden, Allman-Farinelli and Swendseid26 and vice versaReference Selhub, Seyoum, Pomfret and Zeisel24, inverse relations between betaine and homocysteine during folate deficiencyReference Holm, Ueland and Vollset8, and increased betaine concentrations after folic acid supplementationReference Melse-Boonstra, Holm, Ueland, Olthof, Clarke and Verhoef9. The latter findingsReference Melse-Boonstra, Holm, Ueland, Olthof, Clarke and Verhoef9 are in line with results of the present trial.
Conceivably, diseases associated with high plasma tHcy concentrations may not only be linked to low concentrations of cobalamin and folate, but also with low concentrations of betaine and choline. So far, only one non-placebo-controlled pilot study in eight patients with Alzheimer's diseaseReference Knopman and Patterson27 have investigated the effect of oral betaine supplementation, and their results were negative. In contrast, the present trial showed that participants with the largest increases in betaine concentrations (third tertile category; change in betaine concentrations >6·88 μmol/l) showed the highest increase in memory performance compared to those in the first and second tertile categories. Given the fact that the changes in betaine and homocysteine concentrations were most pronounced in the group supplemented with cobalamin plus folate, and were related to the increase in erythrocyte folate concentrations (Table 3), it is possible that folate supplementation accounted for the observed cognitive changes. However, of the recently reported trialsReference McMahon, Green, Skeaff, Knight, Mann and Williams13–Reference Durga, van Boxtel and Schouten15, only the FACIT trial has observed a beneficial effect of folate supplementation on memory functionReference Durga, van Boxtel and Schouten15. The inconclusive results among the reported trials could possibly be explained by variations in study durations, sample sizes, characteristics of study population and assessment of cognitive function.
The apparent effect of the increase in betaine concentrations on improved memory function may also reflect greater availability of choline metabolites for synthesis of the neurotransmitter acetylcholine and several phospholipids, such as phosphatidylcholine and sphingomyelinReference Zeisel28. Choline metabolism is closely related to several aspects of the central nervous system function and structure. An adequate supply of choline appears to be essential for fetal cholinergic neuronal development, in particular for brain regions involved in learning and memory processesReference Zeisel28. Choline is a precursor of brain sphingomyelin, which is a component of white matter myelinReference Zeisel7. It has been proposed that changes in white matter sphingomyelin or phospholipid content precede clinical deterioration in demyelinating diseases such as multiple sclerosisReference Tartaglia, Narayanan and De Stefano29. There is some evidence that supplementation with cytidinediphospho-choline could protect against and prevent memory impairment in ageing ratsReference Teather and Wurtman30, Reference Teather and Wurtman31, and has beneficial effects on memory and behaviour in elderly people with cognitive problemsReference Fioravanti and Yanagi32. The present study describes the association of plasma choline with cognitive status, and the absence of associations prior to supplementation is inconsistent with the hypothesis that choline status is related to cognitive impairment. The lack of associations between plasma choline and cognitive function in the present study may reflect the possibility that plasma free choline represents only a minor fraction of the total choline poolReference Zeisel7, and may be a poor marker of choline status and metabolism in the brain, which has also been previously suggestedReference Amenta, Parnetti, Gallai and Wallin33.
For DMG, participants in the second and third tertile had a better memory performance compared to those in the first tertile. This observation may reflect changes in cognition during altered betaine metabolism because there is a positive relation between the two metabolites at low but not high DMG concentrationsReference Holm, Ueland and Vollset8. However, the DMG–memory association could be a chance finding. This possibility is strengthened by the observation that DMG did not change during the intervention.
In summary, the present trial is the first to explore associations of cognitive function with choline, betaine and DMG. We observed associations of homocysteine and betaine with cognitive domains prior to B-vitamin supplementation. Furthermore, there was a tendency that participants with the largest increases in betaine concentrations due to combined supplementation with cobalamin plus folic acid showed the greatest improvement in memory performance.
Acknowledgements
We are indebted to the participants who took part in this study, and to the directors and staff of the care facility homes for their support. We thank OveÅrseth and Randi Mjelde Heimdal for carrying out all biochemical assays at the LOCUS of Homocysteine and Related Vitamins in Bergen, Lisette Verhoeven, Daniëlle van Hout, Rubia Bloo and Liesbeth Joosten of the Medical Psychology department in Nijmegen for the design of the neuropsychological test battery and their assistance in neuropsychological sessions. In addition, we thank the research assistants, MSc students in Human Nutrition and Neuropsychology, and nurses for their assistance in recruitment and blood collections. This research was supported by grant 2100.0067 from ZON-MW, the Hague, The Netherlands; grant 001-2002 from Kellogg's Benelux, Zaventem, Belgium; grant QLK3-CT-2002-01 775 from the Foundation to Promote Research into Functional Cobalamin Deficiency and the European Union BIOMED Demonstration Project; and grant 2004-E2 from the Nutricia Health Foundation, Wageningen, The Netherlands.