Breast-feeding is a matter of interest as it has been associated with benefits on different aspects of human health. These cover a broad spectrum of immediate effects, such as the maturation of intestinal epithelium(Reference Holscher, Bode and Tappenden1,Reference Turfkruyer and Verhasselt2) and the immune system in the newborns(Reference Boix-Amorws, Puente-Sánchez and du Toit3,Reference Parigi, Eldh and Larssen4) , the decrease in mortality due to infectious diseases, especially in countries with lower income(5). Long-term effects also include, among others, a lower probability of obesity in adults(Reference Armstrong and Reilly6,Reference Forbes, Azad and Vehling7) , atopy, asthma and hypertension(Reference Roberts8), an increase in the intelligence quotient and cognition(Reference Victora, Horta and Loret de Mola9) and a delay in senescence(Reference Kibe, Kurihara and Sakai10). However, concerns exist about some of the long-term benefits of breast-feeding (which were based on observational studies that might have been overstated(Reference Evenhouse and Reilly11–Reference Raissian and Su15)) as well as whether it should be maintained at all costs(Reference Pilkington16).
During the first 6 months, breast-feeding is associated with health advantages compared with infant formulas, which have encouraged the promotion of breast-feeding as the ‘gold standard’ to cover the newborn’s integral necessities(17,Reference McInnes and Stone18) . This fact gives relevant search for crucial components to identify the proper alternative feeds in case breast-feeding is unfeasible. Of the multiple lines of interest, attention has been given to the role of polyamines contained in breast milk. These are ubiquitous polycationic compounds present in all living cells. In mammals, they are synthesised from ornithine being converted to putrescine, by means of ornithine decarboxylase, which is then enzymatically converted to spermidine and then to spermine. The dietary supply of polyamines is also important to maintain essential physiological functions(Reference Larque, Sabater-Molina and Zamora19).
Polyamines stimulate the growth and healing of the gastrointestinal mucosa in animal models(Reference Buts, De Keyser and Kolanowski20–Reference Gao, Guo and Huang23). When they were introduced to milk during lactation, the time of intestinal mucosa proliferation was shortened(Reference Harada, Hashimoto and Syuto24–Reference Wang, Li and Tan27), with an effect on the immune system(Reference ter Steege, Buurman and Forget28,Reference Perez-Cano, Gonzalez-Castro and Castellote29) , supporting their role in the maturation of the intestinal barrier during the neonatal period. Besides trophic effects, polyamines and biogenic primary amines present in the intestinal content modulate spontaneously initiated gastrointestinal motility in the smooth muscle/interstitial cells of Cajal/platelet-derived growth factor receptor-positive cells (SIP) syncytium(Reference Sanchez, Suarez and Andres30). This may facilitate gastric emptying and drive segmentation and peristalsis of the intestine(Reference Sanchez, Suarez and Andres30,Reference Kim, Sim and Choi31) , having a complementary role in the digestion and absorption of nutrients in the microvilli. But neither the specific cellular location (SIP syncytium, enteric innervation and/or smooth muscle cells) for the functional effects of biogenic amines nor the specific chemosensory system receptors have yet been identified. However, non-olfactory trace amine-associated receptors are expressed in the intestine(Reference Borowsky, Adham and Jones32).
Based on its composition, breast milk has been classified into three stages: colostrum, transitional and mature milk(Reference Gidrewicz and Fenton33), with variations in the concentration of polyamines(Reference Plaza-Zamora, Sabater-Molina and Rodriguez-Palmero34); all of which are higher than the content in infant formulas. A wide range of polyamine concentrations exists in human breast milk between different mothers depending on several factors, such as age, race, obesity(Reference Ali, Strandvik and Palme-Kilander35), type of nutrition(Reference Atiya Ali, Strandvik and Sabel36,Reference Gomez-Gallego, Kumar and Garcia-Mantrana37) , mode of delivery(Reference Gomez-Gallego, Kumar and Garcia-Mantrana37), premature birth(Reference Plaza-Zamora, Sabater-Molina and Rodriguez-Palmero34,Reference Atiya Ali, Strandvik and Sabel36) and mastitis(Reference Perez, Ladero and Redruello38). In the newborns, besides oral intake, biliary and pancreatic exocrine secretion(Reference Loret, Brolet and Pierzynowski39), cell exfoliation and intestinal microbiota(Reference Ardeshir, Narayan and Mendez-Lagares40–Reference Nakamura, Ooga and Matsumoto42) are additional intestinal sources of polyamines, which, in turn, may contribute to the establishment of the microbiota(Reference Gomez-Gallego, Collado and Ilo43–Reference Gomez-Gallego, Garcia Romo and Frias45). Absorption capability also determines the homeostasis of intestinal polyamines(Reference Milovic46), which is mainly carried out in the duodenum and ileum.
Despite the immediate known beneficial enteric effects of polyamines, their intestinal content and of other biogenic amines have not been determined in newborns to establish whether variations exist depending on oral ingestion, and this could be of importance regarding the effects described. Safety concerns limit experimental studies in human, leading us to study whether potential variations in polyamine intake (based on the content in human breast milk among mothers and in infant formulas) and/or on the type of feeding may determine the content in faeces of newborns as a function of time from childbirth to day 30 postpartum.
Experimental methods
Study design and participants
This was initially designed as a cohort study, from the first to the 30th day postpartum, to analyse the content of biogenic amines in the mother’s breast milk (or infant formula) and in the faeces of her child. This was not feasible; thus, an observational study was carried out in independent samples comprising fifty-nine Caucasian women and their children. Of them, thirty-nine provided breast milk and twenty infant formula feeding. They were invited to participate consecutively, as they were giving birth at the Hospital Universitario Central de Asturias in Oviedo (Spain) or when attended by the midwife during visits to the health centre (Centro de Salud Parque-Somiw, Gijwn, Spain), in the weeks established to collect samples, as long as they fulfilled the inclusion criteria. It was not possible to obtain samples of both breast milk and faeces in all cases, since sometimes at the same time of day 1 of the samples was not available.
The proposal of the study was explained to the participants who were previously required to provide signed informed consent. The inclusion criteria for the mothers (giving breast milk or infant formula feeding but not mixed feeding at any time) were age from 20 to 40 years, natural vaginal childbirth, the absence of chronic diseases or any disease 2 weeks before the delivery, non-obese and of at least 38 weeks of pregnancy.
The mean age of the mothers supplying breast milk was 33·44 (se 0·84) years old (n 39) and of those opting for infant formula was 31·08 (se 1·4) years old (n 20). The mean number of deliveries were, respectively, 1·48 (se 0·13) and 1·38 (se 0·18). All were vaginally delivered, with thirty-one (52·5 %) boys and twenty-eight (47·5 %) girls.
The study was approved by the Comité de Ética de la Investigaciwn del Principado de Asturias, Spain (reference 24/2011) and was conducted according to the guidelines of the Declaration of Helsinki.
Samples of human breast milk, infant formulas and faeces of the newborn babies
Samples of human milk were collected in sterile Eppendorf© tubes of 1·5 ml, with the following schedule: the same day of delivery (day of giving birth, considered day 0), the next day or day 1, the 3rd, 5th, 7th, 15th and 30th days after childbirth. The minimum volume considered to determine milk amines was 50 µl. The total samples were n 68. The samples of breast milk were maintained at 4°C while transported to the laboratory and then were preserved at −80°C.
Women who chose infant formula, liquid or powder, were asked for a sample to analyse different brands (n 6).
The samples of newborn faeces (for breast milk n 81 and for infant formula fed n 55) were collected following the same schedule and hours as breast milk samples. For this, the diapers were brought refrigerated to the laboratory, then a sample of faeces of approximately 150 mg was introduced in sterile Eppendorf© tubes in 10 % glycerol to be preserved at −80°C, until they were used. Mother–infant pairs of breast milk-fed newborns were forty-one.
Determination of amines in the different types of milk and newborns faeces by HPLC
The amines were determined using a pre-column derivatisation method, as previously described(Reference Escribano and Legaz47,Reference Velasco, Secades and Bordallo48) . The samples of faeces were homogenised and then centrifuged at 4000 rpm for 3 min at room temperature to obtain 300 µl supernatant. These and the samples of milk were treated with perchloric acid to reach a final concentration of 15·8 % (10 min at 4°C). The extracts were then centrifuged at 10 000 g for 30 min at 4°C, and 0·2 ml supernatants were collected and then neutralised with 0·3 ml of a saturated solution of NaHCO3 and the standard (1·25 µl or 5 µl of 1 mmol solution, respectively for milk or faeces). The samples were dansylated overnight (16 h) with 0·5 ml of a solution containing 5 mg/ml of dansyl chloride in acetone, then proline 100 mg/ml was added and left for 30 min. After one extraction with toluene the organic phase was dried under a nitrogen atmosphere at 42°C and afterwards was resuspended in 0·2 ml acetonitrile (VWR).
The samples were chromatographed in an HPLC (Shimadzu Prominence) using a C18 (2·5 μm, 3·0 × 75 mm) reverse-phase column (XBridge from Waters), at room temperature 22–24°C, and equipped with two LC-20AD pumps, a fluorescence detector (RF-20A), an SIL-20AD HT auto sampler injector at 4°C and a DGU-20A3 degasser. The binary gradient used a flow rate of 0·55 ml/min as follows: 0–0·5 min, 0 % B; 0·5–10 min, 75 % B; 10–11·30 min, 75 % B; 11·30–11·50 min, 0 % B, where solvent A was 45 % of acetonitrile and solvent B was 100 % of acetonitrile. The injection volume was of 5 µl.
The duration of the chromatogram was 14 min. The identification of compounds was performed by means of their retention time (standard: 05.88 h, isoamylamine: 07.04 h, putrescine: 07.38 h, cadaverine: 07.83 h, tyramine: 09.89 h, spermidine: 10.38 h, spermine: 12.41 h) and a wavelength of excitation of 365 nm and of emission of 510 nm. All measurements were made using Shimadzu LCsolution Version 1.25 software. The data were the mean of duplicate injections. Quantification of biogenic amines was performed using 2-hydroxydiaminopropane as an internal standard, being expressed as nmol/ml of breast milk or infant formula or in nmol/mg of faeces.
Chemicals
The following drugs were used: putrescine (tetramethylenediamine), spermidine (N-(3-aminopropyl)-1,4-butanediamine), spermine (N,N′-bis(3-aminopropyl)-1,4-butanediamine), isoamylamine (isopentylamine: 1-amino-3-methylbutane), cadaverine (cadaverine dihydrochloride), tyramine (2-(4-hydroxyphenyl)ethylamine) tyramine hydrochloride (4-(2-aminoethyl)phenol hydrochloride) and 2-hydroxydiaminopropane were purchased from Sigma-Aldrich. The drugs were dissolved in purified water with a resistance of 10–15 MΩ × cm.
Statistical analysis
The sample size was conditioned by the feasibility of recruitment, considering at least four different independent samples for each group of days (n), taken as reference similar articles in this field(Reference Plaza-Zamora, Sabater-Molina and Rodriguez-Palmero34). This minimum size of sample corresponded in each case to an equal number of mother’s breast milk, infant formulas or child’s faeces.
The samples were also grouped as ordinal variables following the interval days of the three stages of breast milk: colostrum from days 1 to 5, transitional from days 6 to 14 and mature from days 15 to 30 postpartum. These intervals were also used to analyse the amine content in the faeces of breast-fed and infant formula-fed newborns.
Exploratory analysis was used to determine whether the distribution of amine values in each group of variables significantly differed from a normal distribution. Kolmogorov–Smirnov and Levene’s test, respectively, were used for the analysis of normality and homoscedasticity of each group of variables, assuming normality if P > 0·05. Since for several groups this was violated, non-parametric statistics were used.
The concentrations of biogenic amines in breast milk and faeces were represented as box and whisker plots for the days of the collected sample. The statistical difference in two independent variables (non-parametric), such as for the differences in a particular amine between breast milk and infant formula, was calculated by means of the Mann–Whitney U test. The difference in the amine values obtained between several independent groups of days in different types of feeding was calculated by means of the Kruskal–Wallis test (pairwise comparison), followed by Mann–Whitney test adjusted by Bonferroni correction for multiple tests. The effect size (r) of the comparison of groups was calculated by means of the following formula: r Z score/√N. The group comparison may result in small (0·1), medium (0·3) or large (0·5) effect(Reference Cohen49).
The mean ratios (and standard errors of the mean) of isoamylamine to putrescine, spermidine, spermine, cadaverine and tyramine were used to represent the data and fitted to an exponential equation to obtain the time constant of the decrease (τ).
The Spearman’s rank correlation (r s) (two tailed) was used to determine the association between different amines contained in breast milk, infant formulas or child faeces as well as whether the content in the type of feeding is related to that determined in the faeces. Binary logistic regression was used to establish the interaction of biogenic amines in faeces as predictors of the type of newborn feeding. For all analyses, values of P ≤ 0·05 were considered as significant. The analysis was performed by means of IBM SPSS Statistics version 24.0 (IBM Corp.).
Results
Content of amines in human breast milk and infant formulas
Data showed that varying concentrations of polyamines ton he day of giving birth, as demonstrated by the Kruskal–Wallis test (H(2) = 12·56, P = 0·002, n 30); putrescine (Fig. 1(A)) is significantly lower than spermine (Fig. 1(C)) (z = −3·5, P = 0·001, r −0·8) (Mann–Whitney tests, with adjusted P values), but not lower than spermidine (Fig. 1(B)). No differences exist between spermidine (Fig. 1(B)) and spermine (Fig. 1(C)). The primary amine, isoamylamine (Fig. 1(D)), was present in breast milk at the highest concentration on the day of giving birth (Kruskal–Wallis test (H(3) = 27·06, P < 0·001, n 40). No other biogenic amines were detected. Pairwise comparisons (Mann–Whitney tests with adjusted P values) showed that the concentration of putrescine (Fig. 1(A)) (z = 5·09, P < 0·001, r 1·14) and spermidine (Fig. 1(B)) (z = 3·35, P = 0·005, r 0·75) were lower than those of isoamylamine (Fig. 1(D)) (Supplementary Table S1).
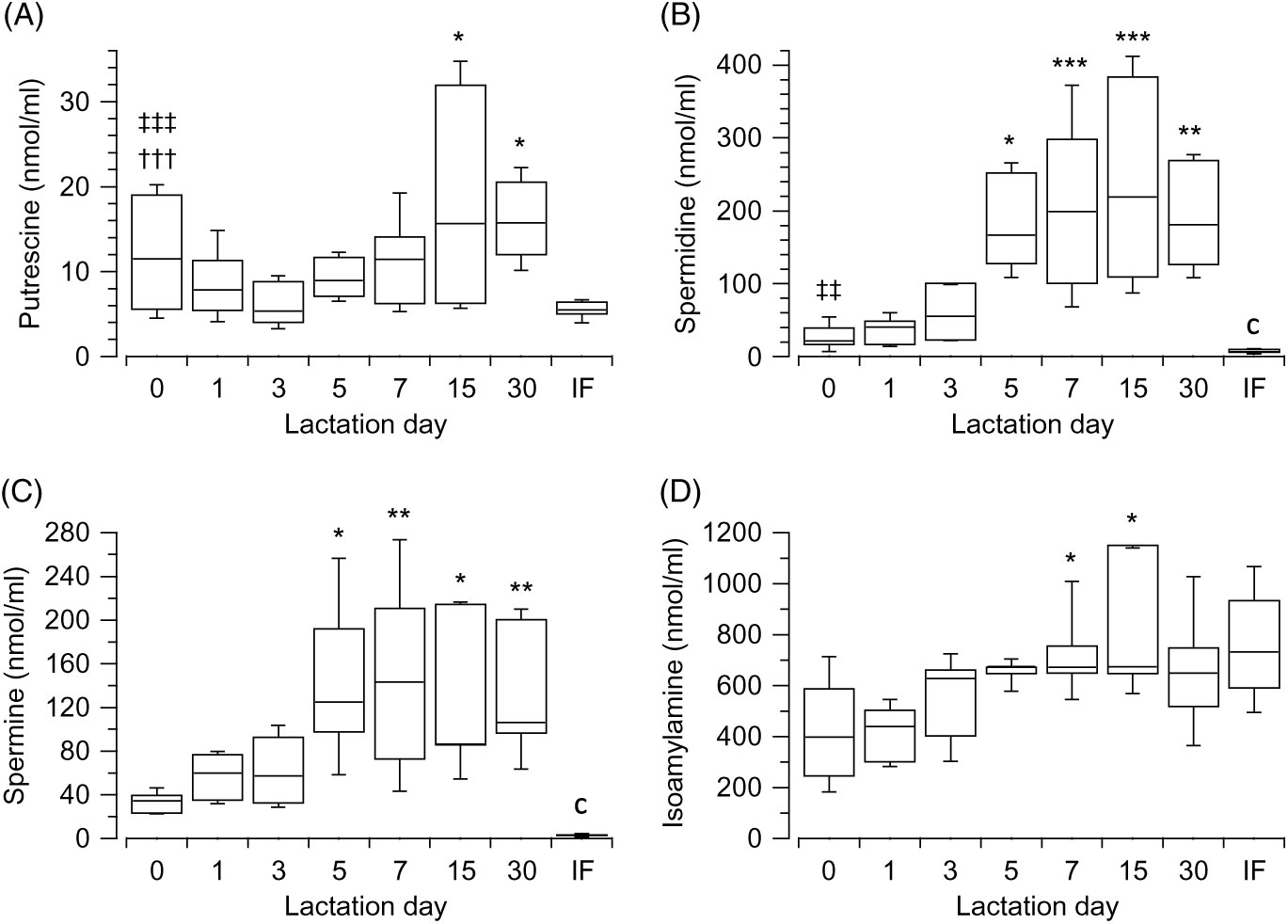
Fig. 1. Box and whisker plots of the values (nmol/ml) of putrescine (A), spermidine (B), spermine (C) and isoamylamine (D) in the breast milk, from the day of giving birth (day 0) to day 30 postpartum. In (A) *P ≤ 0·05 in comparison with day 3; in (B) and (C) *P ≤ 0·05, **P ≤ 0·01 and ***P ≤ 0·001 with respect to the day of giving birth, and in (D) *P ≤ 0·05 day 7 with respect to day 0 and also day 15 with respect to day 1. †††P ≤ 0·001 with respect to spermine, ‡‡ P ≤ 0·01 and ‡‡‡ P ≤ 0·001 with respect to isoamylamine. cP ≤ 0·001 compares infant formulas (IF) with different days of breast milk. P values calculated by non-parametric test.
Polyamines showed an increase during postpartum (from day of giving birth (referred as 0) to day 30) with significant differences in concentrations across the different groups of days, as revealed by the Kruskal–Wallis test: putrescine (Fig. 1(A)) (H(6) = 15·49, P = 0·017, n 66), spermidine (Fig. 1(B)) (H(6) = 42·94, P < 0·001, n 69), spermine (Fig. 1(C)) (H(6) = 27·87, P < 0·001, n 66). Isoamylamine (Fig. 1(D)) equally significantly increased (H(6) = 27·52, P < 0·001, n 68) over time. The comparison (Mann–Whitney tests, with adjusted P values) of concentrations across the groups of days showed that putrescine (Fig. 1(A)) increased significantly when values of day 3 were compared with day 15 (z = −3·21, P = 0·03, r −0·71) and day 30 (z = −3·23, P = 0·024, r −0·77). Spermidine (Fig. 1(B)) increased when values of day 0 were compared with day 5 (z = −3·19, P = 0·029, r −0·82), day 7 (z = −4·14, P = 0·001, r −0·84), day 15 (z = −4·22, P = 0·001, r −0·94) and day 30 (z = −3·77, P = 0·003, r −0·89); and values of day 1 were compared with day 7 (z = −3·92, P = 0·002, r −0·78), day 15 (z = −4·01, P = 0·001, r −0·87) and day 30 (z = −3·56, P = 0·008, r −0·82) and of day 3 to day 15 (z = −3·07, P = 0·046, r −0·69). Spermine (Fig. 1(C)) also increased after day 0 when compared with day 5 (z = −3·28, P = 0·022, r −0·88), day 7 (z = −3·79, P = 0·003, r −0·79), day 15 (z = −3·43, P = 0·013, r −0·81) and day 30 (z = −3·59, P = 0·007, r −0·87). Isoamylamine (Fig. 1(D)) significantly increased when day 0 was compared with day 7 (z = −3·64, P = 0·006, r −0·74) and day 1 with day 7 (z = −4·32, P < 0·001, r −0·94) and with day 15 (z = −3·57, P = 0·008, r −0·84) (Supplementary Table S1).
The values of most amines were higher in breast milk compared with infant formulas, when they were compared with each group of days. A large effect was observed from day 5 for putrescine (Fig. 1(A)) (U = 0, z = −2·56, P = 0·011, r −0·81, n 10) and from day 0 for spermidine (Fig. 1(B)) (U = 4, z = −2·82, P = 0·005, r −0·71, n 16) and spermine (Fig. 1(C)) (U = 0, z = −3·18, P = 0·001, r −0·82, n 15). The values were lower for isoamylamine (Fig. 1(D)) in breast milk compared with infant formula on day 0 (U = 11, z = −2·06, P = 0·039, r −0·52, n 16) and day 1 (U = 5, z = −2·81, P = 0·005, r −0·68, n 17) (Supplementary Table S1).
The analysis of the biogenic amines in the different stages of the breast milk showed differences in the three stages: colostrum (H(3) = 72·16, P < 0·001, n 89), transitional (H(3) = 67·78, P < 0·001, n 82) and mature (H(3) = 50·21, P < 0·001, n 59). Putrescine (Fig. 2(A)) was found to be the least present amine in the three stages when compared with isoamylamine (Fig. 2(D)) (colostrum: z = 8·46, P < 0·001, r 1·27; transitional: z = 8·17, P < 0·001, r 1·29; mature: P < 0·001, r 1·28); spermidine (Fig. 2(B)) (colostrum: z = −3·87, P = 0·001, r −0·58; transitional: z = −4·4, P < 0·001, r −0·7; mature: z = −4·09, P < 0·001, r −0·75); and spermine (Fig. 2(C)) (colostrum: z = −4·58, P < 0·001, r −0·69; transitional: z = −3·54, P = 0·002, r −0·56); mature: z = −2·82, P = 0·029, r −0·52). Spermidine (Fig. 2(B)) (colostrum: z = 4·68, P < 0·001, r 0·706; transitional: z = 3·87, P = 0·001, r 0·597; mature: z = 2·89, P = 0·023, r 0·53) and spermine (Fig. 2(C)) (colostrum: z = 3·88, P = 0·001, r 0·578; transitional: z = 4·75, P < 0·001, r 0·73; mature: z = 4·04, P < 0·001, r 0·75) were also lower than isoamylamine (Fig. 2(D)) (Supplementary Table S2).
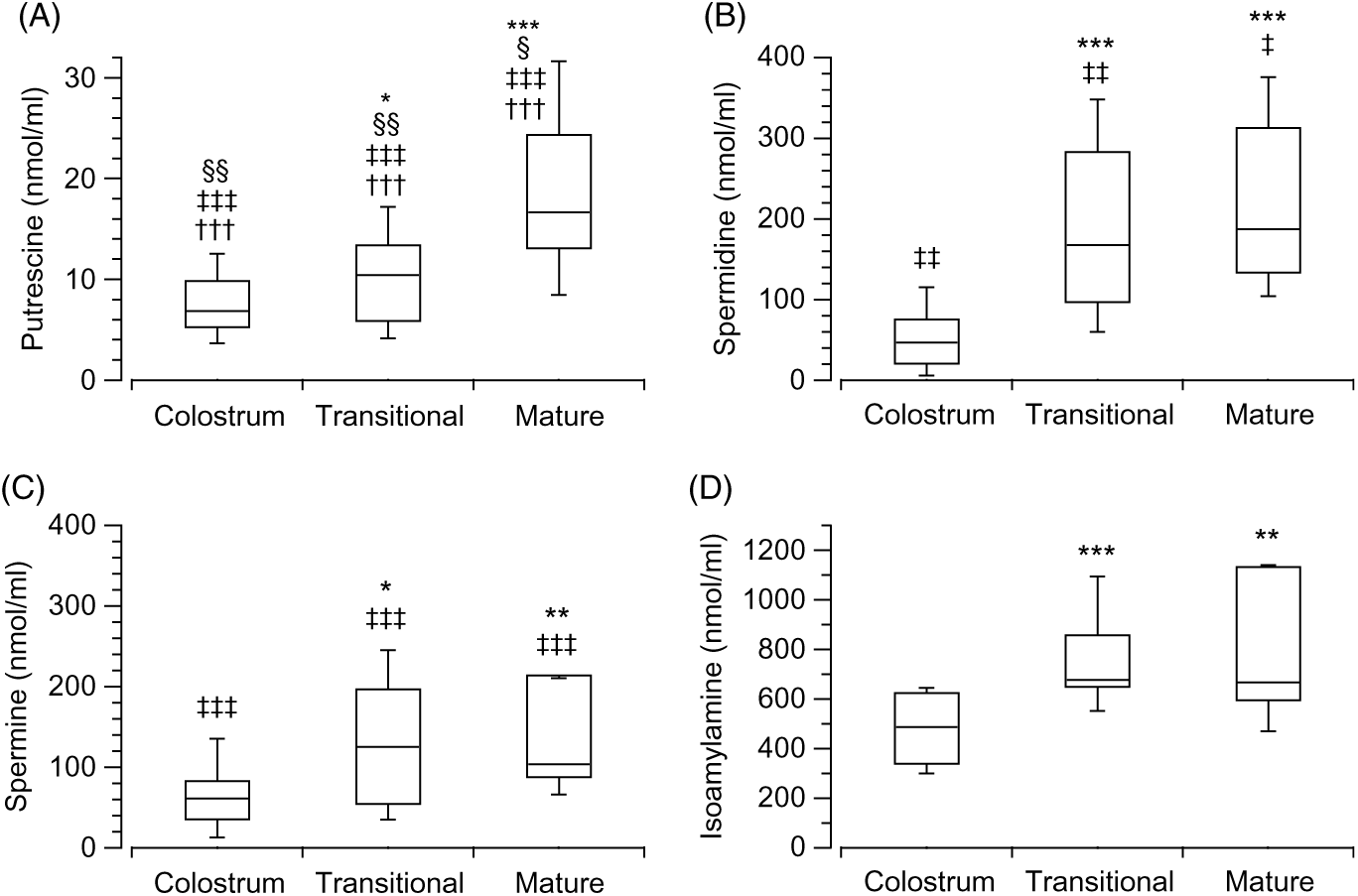
Fig. 2. Box and whisker plots of the values (nmol/ml) of putrescine (A), spermidine (B), spermine (C) and isoamylamine (D) in the stages of breast milk (colostrum, transitional and mature). *P ≤ 0·05, **P ≤ 0·01 and ***P ≤ 0·001 in comparison with colostrum; ‡‡ P ≤ 0·01 and ‡‡‡ P ≤ 0·001 with respect to isoamylamine; †††P ≤ 0·001 with respect to spermidine and §P ≤ 0·05 and §§P ≤ 0·01 with respect to spermine on the same stage. P values calculated by non-parametric test.
The values of each amine were analysed (Kruskal–Wallis test) to determine the change during the various breast milk stages. This showed significant differences between the stages (colostrum, transitional and mature): putrescine (Fig. 2(A)) (H(2) = 15·95, P < 0·001, n 56), spermidine (Fig. 2(B)) (H(2) = 27·25, P < 0·001, n 59), spermine (Fig. 2(C)) (H(2) = 10·57, P = 0·005, n 57) and isoamylamine (Fig. 2(D)) (H(2) = 20·62, P < 0·001, n 58). Mann–Whitney tests (pairwise comparisons with adjusted P values) showed that putrescine (Fig. 2(A)) increased in the mature stage when compared with colostrum (z = −3·96, P < 0·001, r −0·65) and transitional stages (z = −2·73, P = 0·019, r −0·47), spermidine (Fig. 2(B)) increased in the transitional (z = −4·08, P < 0·001, r −0·62) and mature stage (z = −4·7, P < 0·001, r −0·76) when compared with colostrum; and the concentrations of spermine (Fig. 2(C)) also increased in the transitional (z = −2·44, P = 0·044, r −0·37) and mature stages (z = −3, P = 0·008, r −0·5) when compared with colostrum. Isoamylamine (Fig. 2(D)) concentrations were also higher in the transitional (z = −4·33, P < 0·001, r −0·66) and the mature stage (z = −3·15, P = 0·005, r −0·52) compared with the colostrum stage (Supplementary Table S2).
Correlation between the amines present in human breast milk or infant formulas
In breast milk, the values of putrescine correlated positively and significantly with spermidine (r s = 0·53, P < 0·001, n 65) and spermine (r s = 0·54, P < 0·001, n 62), and the values of spermidine correlated positively and significantly with spermine (r s = 0·93, P < 0·001, n 65). The concentration of isoamylamine correlated positively and significantly with spermidine (r s = 0·55, P < 0·001, n 67) and spermine (r s = 0·4, P < 0·001, n 64). In infant formulas, no significant correlations exist between the different amines.
Content of biogenic amines in the faeces of newborns
In the meconium (day 0), no differences were observed in the content of each polyamines depending on the type of feeding (Fig. 3). Although differences were observed when the content of polyamines were compared in each type of feeding (breast-fed: H(2) = 37·98, P < 0·001, n 60; infant formula feed: H(2) = 27·85, P < 0·001, n 42). In both cases, putrescine (Fig. 3(A)) was the polyamine present at the highest concentration (Mann–Whitney tests with adjusted P values), showing large effect size when compared with spermidine (Fig. 3(B)) (z = 5·38, P < 0·001, r 0·85 and z = 4·88, P < 0·001, r 0·92, respectively, for each type of feeding) and spermine (Fig. 3(C)) (z = 5·3, P < 0·001, r 0·83 and z = 4·18, P = 0, r 0·79, respectively, for each type of feeding). Considering all the amines, the content of isoamylamine (Fig. 3(D)) was higher than putrescine (Fig. 3(A)) in breast milk feeding (U = 66, z = −3·63, P < 0·001, r −0·57, n 40) and in infant formula feeding (U = 40, z = −2·67, P = 0·008, r −0·5, n 28). No differences were observed in the content of isoamylamine (Fig. 3(D)), cadaverine (Fig. 3(E)) and tyramine (Fig. 3(F)) between both types of feeding.
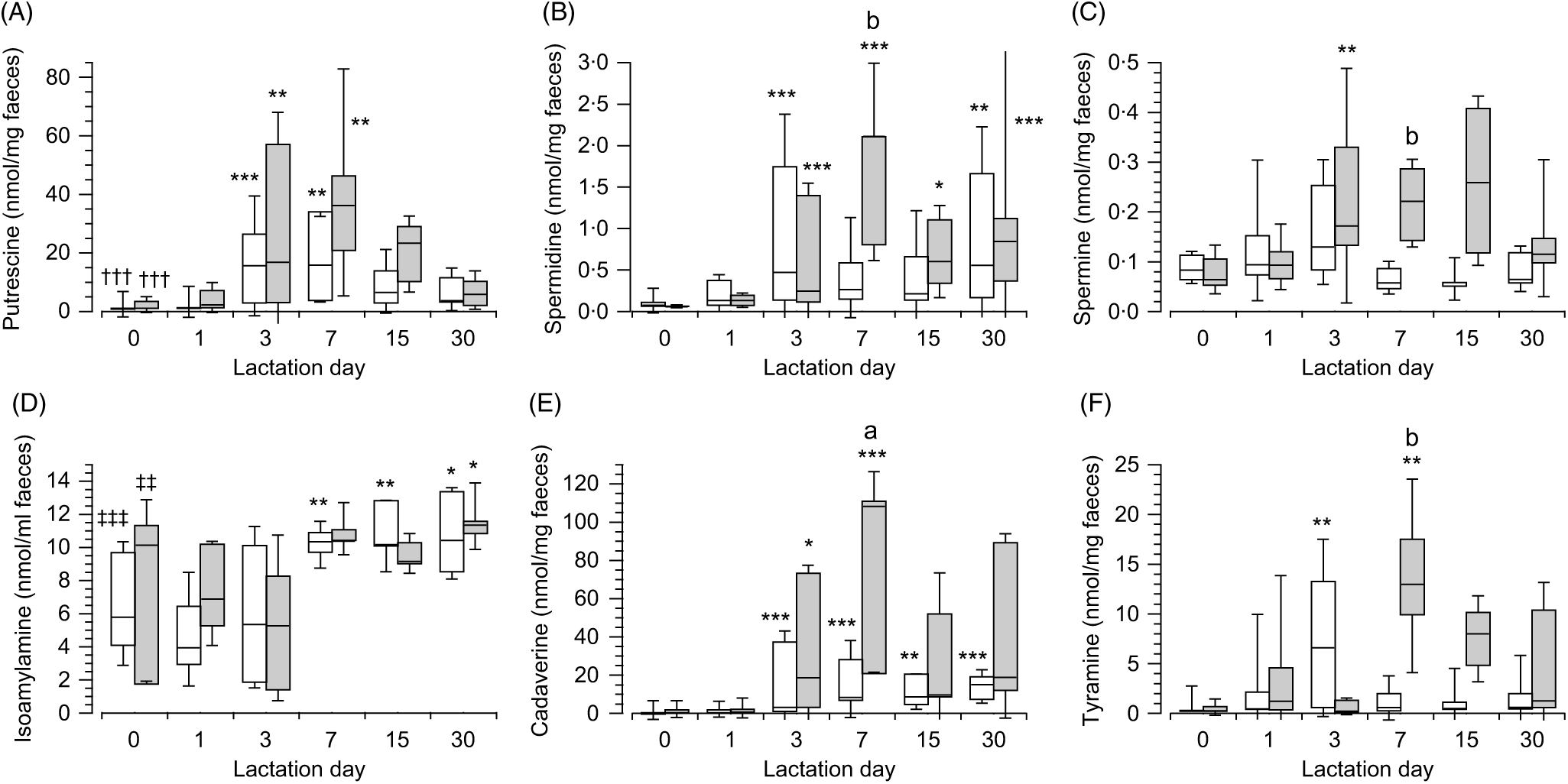
Fig. 3. Box and whisker plots of the values in nmol/mg of faeces for putrescine (A), spermidine (B), spermine (C), isoamylamine (D), cadaverine (E), and tyramine (F) in breast (white) or infant formula (grey) fed from the day of birth (day 0) to day 30 postpartum. *P ≤ 0·05, **P ≤ 0·01 and ***P ≤ 0·001 in comparison with day 0; †††P ≤ 0·001 with respect to spermidine and spermine and ‡‡P ≤ 0·01 and ‡‡‡P ≤ 0·001 isoamylamine with respect to the rest of the amines. aP ≤ 0·05 and bP ≤ 0·01 compare the content of amines in the faeces of infant formula-fed newborns with breast milk-fed newborns. P values calculated by non-parametric test. , Breast milk;
, infant formula.
The concentrations of polyamines in faeces increased over time, with significant differences across the groups of days in both types of feeding (expressed for breast-fed and infant formula-fed, respectively, putrescine (Fig. 3(A)): H(5) = 28·94, P < 0·001 (n 79) and H(5) = 22·84, P < 0·001 (n 55), spermidine (Fig. 3(B)): H(5) = 24·96, P < 0·001 (n 79) and H(5) = 25·79, P < 0·001 (n 55), and spermine (Fig. 3(C)): H(5) = 18·07, P = 0·003 (n 79) and H(5) = 18·52, P = 0·002 (n 55)). Pairwise comparisons (Mann–Whitney tests with adjusted P values) of polyamines in the faeces of breast milk-fed newborns showed that putrescine (Fig. 3(A)) content was highest on day 3 (z = −3·91, P = 0·001, r −0·66) and day 7 (z = −3·61, P = 0·004, r −0·68) from the start of breast-feeding as well as for these days when compared with the 1st day (z = −3·54, P = 0·006, r −0·6 and z = −3·3, P = 0·014, r −0·64, respectively). The values of spermidine (Fig. 3(B)) were highest on day 3 (z = −4·04, P < 0·001, r −0·68) and day 30 (z = −3·6, P = 0·004, r −0·71) when compared with day 0. No significant differences were observed for spermine (Fig. 3(C)) across the groups of days. Similarly, in newborns fed with infant formula the concentration of putrescine (Fig. 3(A)) was highest on day 3 (z = −3·57, P = 0·005, r −0·65) and day 7 (z = −3·62, P = 0·004, r −0·83) after birth with respect to day 0, which decreased subsequently. Spermidine (Fig. 3(B)) increased on day 3 (z = −4, P < 0·001, r −0·73) and also significantly increased on days 7 (z = −4·41, P < 0·001, r −1·01), 15 (z = −3·16, P = 0·023, r −0·75) and 30 (z = −3·93, P = 0·001, r −0·9) when compared with day 0 and increased on day 7 when compared with day 1 (z = −3·12, P = 0·027, r −0·78). Spermine (Fig. 3(C)) also increased on day 3 (z = −3·49, P = 0·007, r −0·64) when compared with day 0 (Supplementary Tables S3 and S4).
As for polyamines, the content of the primary amines in the faeces of newborns varied over time after birth in both types of feeding (isoamylamine (Fig. 3(D)): H(5) = 24·02, P < 0·001 (n 79, and H(5) = 11·58, P = 0·041 (n 55); cadaverine (Fig. 3(E)): H(5) = 33·92, P < 0·001 (n 79), and H(5) = 26·25, P < 0·001 (n 54); tyramine (Fig. 3(F)): H(5) = 14·8, P = 0·011 (n 79), and H(5) = 15·99, P = 0·007 (n 51), respectively, for breast milk and infant formula feeding). Pairwise comparison between groups of days in breast milk-fed newborns (Mann–Whitney tests with adjusted P values) showed that isoamylamine (Fig. 3(D)) increased on day 7 (z = −3·22, P = 0·019, r −0·62) after birth and maintained nearly the same levels until day 15 (z = −3·44, P = 0·008, r −0·68) and day 30 (z = −3·28, P = 0·015, r −0·66). Cadaverine (Fig. 3(E)) increased on day 3 (z = −3·94, P = 0·001, r −0·74) and remained at around the same level on day 7 (z = −4·02, P < 0·001, r −0·76), day 15 (z −3·74, P = 0·002, r = −0·63) and day 30 (z = −3·98, P = 0·001, r −0·78) after birth. Tyramine (Fig. 3(F)) showed a peak increase on day 3 (z = −3·78, P = 0·002, r −0·64) with respect to day 0. For infant formula-fed newborns (Mann–Whitney tests with adjusted P values), isoamylamine (Fig. 3(D)) was significantly highest on day 30 (z = −3·08, P = 0·02, r −0·67) with respect to day 3 after birth; cadaverine (Fig. 3(E)) increased after day 3 (z = −3·34, P = 0·012, r −0·61), peaked on days 7 (z = −3·89, P = 0·001, r −0·89) and 30 (z = −2·97, P = 0·044, r −0·68) when compared with day 0 and also peaked on day 7 (z = −3·29, P = 0·014, r −0·82) when compared with the 1st day after birth. Tyramine (Fig. 3(F)) showed a peak increase on day 7 (z = −3·46, P = 0·008, r −0·79) when compared with the day of birth (Supplementary Tables S3 and S4).
The magnitude of increase in the biogenic amines in faeces, over time, were significantly higher for infant formula-fed newborns compared with breast milk-fed newborns on day 7, namely, spermidine (Fig. 3(B)) (U = 5, z = −2·45, P = 0·014, r −0·63, n 15), spermine (Fig. 3(C)) (U = 2, z = −2·82, P = 0·005, r −0·73, n 15), cadaverine (Fig. 3(E)) (U = 8, z = −2·08, P = 0·037, r −0·54, n 15) and tyramine (Fig. 3(F)) (U = 4, z = −2·57, P = 0·01, r −0·66, n 15) and also for tyramine (Fig. 3(F)) at day 15 (U = 3, z = −2·32, P = 0·021, r −0·64, n 13). No statistical significance was shown for putrescine (Fig. 3(A)), whose differences were NS, and for isoamylamine (Fig. 3(D)), which was very similar in both types of feeding (Supplementary Tables S3 and S4).
The biogenic amine content in faeces was also analysed in the intervals of days considered to establish the breast milk stages (colostrum from days 1 to 5, transitional from days 6 to 14 and mature from days 15 to 30 after birth). The comparison dependent on the type of feeding showed no differences during the days of colostrum. However, during transitional stage days, spermidine (U = 11, z = −2·39, P = 0·016, r −0·52, n 21), spermine (U = 11, z = −2·39, P = 0·016, r −0·52, n 21), cadaverine (U = 12, z = −2·31, P = 0·02, r −0·5, n 21) and tyramine (U = 9, z = −2·56, P = 0·01, r −0·56, n 21) were higher in faeces of infant formula-fed newborns compared with those fed breast milk. Spermine (U = 26, z = −2·83, P = 0·004, r −0·54, n 27) and tyramine (U = 41, z = −2·06, P = 0·039, r −0·39, n 27) were still higher in the faeces of those infant formula fed during the days of mature stage (Supplementary Table S5).
The content of the biogenic amines in faeces varied significantly across the days of the stages, depending on the type of feeding: spermine in breast-fed (H(2) = 10·09, P = 0·006, n 68) and spermidine in the infant formula fed (H(2) = 12·38, P = 0·002, n 41), and isoamylamine in both types (H(2) = 16·96, P < 0·001, n 68 and H(2) = 12, P = 0·002, n 41, respectively) and cadaverine (H(2) = 8·89, P = 0·012, n 68 and H(2) = 8·63, P = 0·013, n 41, respectively). In the faeces of breast-fed newborns, the content of spermine decreased significantly in the mature stage days when compared with colostrum days (z = 2·94, P = 0·01, r 0·407), while isoamylamine significantly increased in the transitional (z = −2·65, P = 0·024, r −0·37) and mature stage days (z = −3·86, P < 0·001, r −0·53) with respect to colostrum as well as cadaverine in the mature stage days (z = −2·68, P = 0·022, r −0·37). For infant formula-fed newborns, spermidine increased in transitional (z = −2·95, P = 0·01, r −0·52) and mature (z = −2·47, P = 0·041, r −0·41) stages with respect to colostrum days and also increased isoamylamine in the transitional (z = −2·38, P = 0·05, r −0·4) and mature stage days (z = −2·94, P = 0·01, r −0·52) and cadaverine in the transitional stage days (z = −2·74, P = 0·019, r −0·48). No significant modifications were observed for the remaining biogenic amines (Supplementary Table S5).
Ratios of isoamylamine with the other amines in faeces of both types of feeding
The finding that isoamylamine content and variations over time were similar in the faeces of both types of feeding led us to use this amine as a reference to normalise the other amines as a ratio. All the ratios decreased, with an analogous time constant (1·64 ± 0·16 d), from the day after birth until day 5, without significant differences between breast milk- and infant formula-fed newborns (Fig. 4). Then the ratios of isoamylamine:spermidine (Fig. 4(B)) (U = 5, z = −2·45, P = 0·014, r −0·63, n 15), isoamylamine:spermine (Fig. 4(C)) (U = 2, z = −2·82, P = 0·005, r −0·73, n 15), isoamylamine:cadaverine (Fig. 4(D)) (U = 8, z = −2·08, P = 0·037, r −0·54, n 15) and isoamylamine:tyramine (Fig. 4(E)) (U = 4, z = −2·57, P = 0·01, r −0·66, n 15) increased significantly on day 7 but not of isoamylamine:putrescine (Fig. 4(A)). The ratios of isoamylamine:spermine (Fig. 4(C)) (U = 2, z = −2·47, P 0·014, r −0·68, n 13) and isoamylamine:tyramine (Fig. 4(E)) (U = 3, z = −2·32, P = 0·021, r −0·64, n 13) remained in the same level on day 15.

Fig. 4. Ratios of isoamylamine concentration to putrescine (A), spermidine (B), spermine (C), cadaverine (D), or tyramine (E) in the faeces of breast milk-fed () and infant formula-fed (
) newborns, from the day of birth (day 0) to day 30 postpartum. Values are means, with standard errors represented by vertical bars. aP ≤ 0·05 and bP ≤ 0·01 compare infant formulas with different days of breast milk. P values calculated by non-parametric test.
Correlation between biogenic amines in faeces of the newborns fed human breast milk and infant formulas
In the faeces of breast-fed newborns, the content of putrescine correlated positively and significantly with spermidine (r s 0·62, P = 0·001, n 81) and spermidine with spermine (r s = 0·35, P = 0·001, n 81). Putrescine correlated significantly with cadaverine (r s = 0·78, P = 0·001, n 81) and tyramine (r s = 0·52, P = 0·001, n 76). In addition, spermine correlated negatively and significantly with isoamylamine (r s = −0·52, P = 0·001, n 81) and positively with tyramine (r s = 0·51, P = 0·001, n 76). Cadaverine positively correlated with tyramine (r s = 0·51, P = 0·001, n 76) and isoamylamine (r s = 0·24, P = 0·03, n 81).
In infant formula-fed newborns, putrescine correlated significantly with spermidine (r s = 0·7, P < 0·001, n 55) and spermine (r s = 0·52, P < 0·001, n 55), and spermidine with spermine (r s = 0·7, P < 0·001, n 55). Putrescine, spermidine and spermine correlated positively with cadaverine (respectively, r s = 0·81, P < 0·001, n 54; r s = 0·73, P < 0·001, n 54; and cadaverine r s = 0·52, P < 0·001, n 54) and tyramine (respectively, r s = 0·64, P < 0·001, n 51; r s = 0·64, P < 0·001, n 51; and r s = 0·58, P < 0·001, n 51). Cadaverine correlated positively with tyramine (r s = 0·49, P < 0·001, n 50).
Correlation between polyamines and primary amines in faeces of the newborns with respect to the type of feeding, breast milk or infant formula feeding
In breast-fed newborns, the concentration of putrescine in breast milk correlated positively with the content of isoamylamine in faeces (r s = 0·42, P = 0·007, n 40). The concentration of spermidine in breast milk correlated positively with the content of putrescine (r s = 0·46, P = 0·002, n 42), spermidine (r s = 0·43, P = 0·005, n 42), isoamylamine (r s = 0·58, P < 0·001, n 42) and cadaverine (r s = 0·47, P = 0·002, n 42) in faeces. Similarly, spermine in breast milk correlated with cadaverine in faeces (r s = 0·43, P = 0·005, n 40). Also isoamylamine in breast milk correlated positively with the content in faeces of this amine (r s = 0·59, P < 0·001, n 41) and of putrescine (r s = 0·4, P = 0·01, n 41), cadaverine (r s = 0·41, P = 0·008, n 41), spermidine (r s = 0·36, P = 0·023, n 41) and spermine (r s = −0·47, P = 0·002, n 41).
In faeces of infant formula-fed newborns, the content of isoamylamine in faeces correlated positively with the concentration of putrescine (r s = 0·4, P = 0·002, n 55) and spermidine (r s = 0·53, P < 0·001, n 55) in the breast milk.
When the analysis was restricted to the days included in the transitional and mature stages, the content of spermine in faeces of breast-fed newborns correlated positively with the concentration of spermidine (r s = 0·47, P = 0·037, n 20) and spermine (r s = 0·45, P = 0·048, n 20) in breast milk. Also in the infant formula-fed newborns, the isoamylamine (r s = 0·6, P = 0·025, n 14) and tyramine (r s = −0·61, P = 0·022, n 14) contents in faeces were correlated positively and negatively, respectively, with the concentration of spermidine in the infant formulas.
Prediction of breast milk or infant formula feeding based on faeces amine content
Regression logistic analysis was used to determine the possibility of using amines to predict the types of newborn feeding, for the days included in transitional and mature stages where differences in amines were found. The model contains two independent variables cadaverine and tyramine in nmol/mg of faeces, statistically significant χ 2 (2, n 48) = 27·47, P < 0·001, indicating that the model was able to distinguish between breast milk and infant formula. The model correctly classified 85·4 % of the cases (Cox and Snell R 2: 34·7 % and Nagelkerke R 2: 36·3 %). Only two of the independent variables made a unique statistically significant contribution to the model (cadaverine and tyramine in faeces). The strongest predictor of the feeding type was the content of tyramine in faeces, recording an OR of 1·29, then cadaverine in faeces, 1·027·The formula to predict the type of feeding was Y breast milk/infant formula = −2·837 + (cadaverine (nmol/mg faeces) × 0·026) + (tyramine (nmol/mg faeces) 0·258), with a cutoff value of 0·5.
Discussion
The results of the study showed that the contents of polyamines and primary amines in the faeces of newborns, during the first 30 d after birth, are determined by the feeding type, that is, breast milk or infant formula, but it is not related to the amount ingested. The differences in the content may be related to the distinct presence of amine-producing bacteria, which polyamines can help establish.
The first and only source of nutrition in newborns is breast milk or, alternatively, feeding with infant formulas. Both feeding types provide several components, polyamines being one of them, which crucial to the development of the gastrointestinal tract and the establishment of microbiota and immune system, with difficult results. Consequently, it is a matter of interest to determine the influence of variations in the amounts of polyamines provided on their intestinal content (subsidiary studied in faeces).
The measurement of polyamines in human breast milk showed that at birth and for the first 3 d the most concentrated were spermidine and spermine. All then increased up to the 2nd week postpartum, with a tendency to decrease afterwards. Spermidine was the most concentrated polyamine, reaching roughly four to five times the initial concentration. When referring to the stages of breast milk, the concentrations of polyamines were lower in the colostrum stage, putrescine being the least concentration, and then increased in the transitional and mature stages. Overall, these data are in line with previous reports, showing increases in polyamines over time, though quantitative differences are present(Reference Plaza-Zamora, Sabater-Molina and Rodriguez-Palmero34,Reference Pollack, Koldovsky and Nishioka50,Reference Romain, Dandrifosse and Jeusette51) . These have been explained by the lifestyle, type of diet, the characteristics of the women and perinatal factors, as mentioned above, and by the different methods used to extract the biogenic amines of the samples among diverse studies. To minimise the influential factors as much as possible, our study was performed in the same geographical area on Caucasian, non-obese, healthy women, who gave birth after the 38th week of pregnancy to vaginally delivered infants (to avoid differences in the polyamines and of the acquired microbiota(Reference Gomez-Gallego, Kumar and Garcia-Mantrana37,Reference Dominguez-Bello, De Jesus-Laboy and Shen52–Reference Cabrera-Rubio, Mira-Pascual and Mira54) ), and using both types of feeding methods. The study demonstrated, as has previously been observed, the existence of individual variations in polyamine content (including spermine, in some studies less susceptible to environmental influences(Reference Gomez-Gallego, Kumar and Garcia-Mantrana37,Reference Kumar, du Toit and Kulkarni55) ) found in the breast milk of different mothers, at least partly related to different human milk microbiota profiles(Reference Gomez-Gallego, Kumar and Garcia-Mantrana37,Reference Pannaraj, Li and Cerini56,Reference Timmerman, Rutten and Boekhorst57) . It should be noted that despite the similar characteristics and socio-cultural environment of the mothers and expecting similar profile in breast milk microbiota within the geographical area(Reference Urbaniak, Angelini and Gloor53,Reference Kumar, du Toit and Kulkarni55,Reference Pannaraj, Li and Cerini56) , they could have had dietary habits (not investigated in the study) that may have affected the content of polyamines in breast milk, which may have had physiological consequences.
The comparison of the polyamine content in both types of feeding showed that spermidine and spermine were significantly lower in infant formulas compared with breast milk for the entire 1st month. For putrescine, the changes were observed after the 1st week postpartum, similar to previous reports(Reference Pollack, Koldovsky and Nishioka50,Reference Romain, Dandrifosse and Jeusette51,Reference Buts58,Reference Dorhout, van Beusekom and Huisman59) . In breast milk, but not in infant formulas, there existed linear positive correlations between putrescine and spermidine, between putrescine and spermine and between spermidine and spermine. These correlations may reflect common sources of production. Infant formulas are given at a fixed composition of constituents, which does not match the variations over time observed in breast milk(Reference Romain, Dandrifosse and Jeusette51).
The primary amine isoamylamine was identified in breast milk and infant formulas, and no other biogenic amines were present. In infant formula biogenic amines were infrequently detected or were present at very low levels(Reference Spizzirri, Puoci and Lemma60). Its presence is associated with poor hygienic quality in non-fermented foods(Reference Barbieri, Montanari and Gardini61). Therefore, this indicates that our samples were correctly preserved. It is interesting that isoamylamine in breast milk also varies with time, increasing until the 1st week after delivery. The first days were less concentrated than in infant formulas and, subsequently, were similar. Isoamylamine presence in breast milk and its sources have not been previously mentioned, its sources are also unknown in other human tissues where it has been found(Reference Meana, Rubin and Bordallo62). It has been reported in mice kidney that expressed a specific decarboxylase of leucine forming isoamylamine(Reference Lambertos, Ramos-Molina and Cerezo63) and is also produced by bacteria(Reference Sutton and King64–Reference Delbes-Paus, Pochet and Helinck67). Among them, Lactobacillus plantarum (considered a probiotic species by the European Food Safety Authority) and Enterococcus faecalis are present in breast milk(Reference Soto, Martin and Jimenez68–Reference Jost, Lacroix and Braegger70). These bacteria produce isoamylamine, at least in cheese(Reference Delbes-Paus, Pochet and Helinck67). In breast milk, but not in infant formulas, isoamylamine correlated positively with the concentrations of spermidine and spermine, suggesting the existence of producer or degrader factors in common with the polyamines, affecting or being affected by them. This matter has not yet been studied in human milk.
The determination of polyamines and primary amines in the meconium of newborns revealed that at birth no differences were observed in the content of amines between the two feeding groups. This appears to demonstrate their homogeneity, which was taken into consideration when choosing term pregnancies by giving natural birth, which may affect newborns’ microbiota(Reference Urbaniak, Angelini and Gloor53,Reference Akagawa, Tsuji and Onuma71) . The average age and number of deliveries of the mothers and the sex of the newborns were also similar. In the meconium, the most important amine was isoamylamine, and putrescine of the polyamines. Histamine was not present, or could have also been below the detection levels, in the faeces of newborns.
After birth, nutrition is one of the sources of polyamines, contributing to those produced by the organs of the digestive system and the microbiota. For primary amines (except for isoamylamine, present in breast milk), the main source is produced by intestinal bacteria, which may vary depending on the feeding type of newborns(Reference Pannaraj, Li and Cerini56,Reference Timmerman, Rutten and Boekhorst57,Reference Borewicz, Gu and Saccenti72) . Through breast milk, the mother’s microbiota is transferred and helps in establishing the newborns’ own microbiota. The commensal bacteria and the antimicrobial factors produced by the microbiota or by compounds present in human milk (bioactive or acting as probiotics) are important. In addition, the intestinal absorption of polyamines determines its intraluminal content.
Our results showed that in the faeces of newborns, polyamines and the primary amines increased differently from day 3 after birth, the peak amplitude being in infant formula fed on day 7, which is greater than that in breast-fed (except for putrescine, no significant change, and isoamylamine, that followed similar evolution over time in both groups). This did not match the quantitative changes observed over time in breast milk and infant formula. In fact, it was the opposite, suggesting that other factors, apart from nutrition, are involved. However, an association between some polyamines and biogenic amine content seemed to exist in faeces, with differences based on the type of feeding. Thus, during the days of transitional and mature stages of breast milk, positive correlations existed between the content of isoamylamine and tyramine in the faeces with the presumable intake of spermidine by breast milk. While in the faeces of infant formula-fed children, the content of cadaverine correlated significantly to spermine intake, and the content of spermine in faeces with the content of spermidine and spermine in the infant formulas. The analysis of the ratios of isoamylamine to the remaining amines showed that they decreased with a time constant of about 2 d, and after day 5 these were mostly stable, independent of the type of nutrition, suggesting an equilibrium in the homeostatic factors determining their concentrations. The only exceptions were in the faeces of infant formula-fed newborns during the 2nd week; there were an increase in the ratios of isoamylamine:spermidine and, especially, isoamylamine:spermine and isoamylamine:tyramine, in comparison with breast-fed infants. These differences indicate that the homeostases of biogenic amines in the faeces are complex and determined by more factors than the amount ingested. But were reduced by the end of the 1st month.
In adult faecal microbiota, diverse micro-organisms have been associated with the production of biogenic amines depending on the strain and the culture conditions, when grown in the presence of a cocktail of amino acids (not leucine) or amines (polyamines, cadaverine, tyramine and histamine, but not isoamylamine). In the presence of these amines, overall a decrease in the production of putrescine and cadaverine by most Gram-positive bacteria and of tyramine mostly by Enterococcus strains were observed. While increases in the production of spermidine, spermine and histamine in several Gram-positive and Gram-negative strains have been demonstrated(Reference Pugin, Barcik and Westermann73). It is not easy to extrapolate this in vitro experiment, a cocktail with a fixed concentration of amines (0·03 %), with the variable conditions of the nutritional factors(Reference Borewicz, Gu and Saccenti72,Reference Madan, Farzan and Hibberd74) and microbiota(Reference Pannaraj, Li and Cerini56,Reference Timmerman, Rutten and Boekhorst57,Reference Boix-Amoros, Collado and Mira75,Reference Cabrera-Rubio, Collado and Laitinen76) found in newborns during lactation. But it suggests that the ingested polyamines and isoamylamine may contribute to facilitating or limiting the growth of different faecal bacteria strain producers or degraders of biogenic amines. An inter-individual variability exists in the microbiota within breast-fed newborns and between the type of feeding(Reference Timmerman, Rutten and Boekhorst57,Reference Palmer, Bik and DiGiulio77,Reference Penders, Vink and Driessen78) . Among the bacteria associated with infant formula feeding, Streptococcus and Enterococcus species were discriminatory(Reference Timmerman, Rutten and Boekhorst57). Some strains of Enterococcus faecalis are producers of putrescine, tyramine and cadaverine(Reference Pugin, Barcik and Westermann73,Reference Ladero, Fernandez and Calles-Enriquez79,Reference Ladero, Cañedo and Pérez80) , Escherichia coli also produces cadaverine(Reference Pugin, Barcik and Westermann73). The presence of these bacteria in faeces might explain the higher content of putrescine and cadaverine found in infant formula-fed infants.
In addition, human breast milk feeding facilitates the histological and functional maturation of the epithelium and intestinal villi and of the immune system after birth and may also decrease inflammation caused by the introduction of oral feeding in infants(Reference Campeotto, Butel and Kalach81). Among other aspects, polyamines are involved in these effects(Reference van Wettere, Willson and Pain26,Reference Sabater-Molina, Larque and Torrella82) . Intestinal maturation is going to presumably increase the capability of absorption, decreasing the intestinal content of polyamines and of amino acids, of which leucine, lysine and tyrosine are precursors of isoamylamine, cadaverine and tyramine, respectively, altering their concentration in faeces. The greater concentration of polyamines and primary amines in faeces, among other mechanisms, could explain the reduction in the frequency of stools and the alteration in faecal consistency in infant formula feeding. This seems to suggest the decrease in motility shown on segmentation contractions and peristalsis of isolated intestinal tissues(Reference Sanchez, Suarez and Andres30,Reference Kim, Sim and Choi31) (with effects on the intestinal digestion and absorption of nutrients) and the gastrointestinal benefits for children’s diarrhoea, when the solutions for oral rehydration or infant formulas were supplemented with polyamines(Reference Tang, Cheng and Sun83). These effects could be receptor dependent, being involved in the crosstalk between the microbiota and intestinal function(Reference Nicholson, Holmes and Kinross84), since trace amine-associated receptors for cadaverine, isoamylamine and tyramine have been reported in the gut(Reference Borowsky, Adham and Jones32).
During the 2nd week, results showed a quantitative difference in the content of polyamines, cadaverine and tyramine in faeces, depending on the type of feeding. Additional studies should clarify the factors that determine these differences: nutritional, microbiota and/or effects on intestinal maturation and absorption. The concentrations of polyamines during lactation or when giving infant formulas could be adjusted and then evaluated for their influence on the biogenic amines in faeces. The determination of cadaverine and tyramine in faeces may be useful as biological markers to determine the polyamines supplied during the first 2 weeks after birth, since they may predict, with 85 % of accuracy, the type of feeding method given.
It also seems important to determine in faeces the relevant amine or amines, and its concentrations, with the corresponding histological and metabolic gastrointestinal effects as well as identify the micro-organisms that produce the biogenic amines found in the faeces that may provide information about potential differences in the microbiota. In newborns, the experimental studies supplementing polyamines orally should be considered carefully due to safety issues(Reference Pegg85,Reference Sharmin, Sakata and Kashiwagi86) . Although it will be of interest to determine whether supplementing polyamines may result in faeces presenting similar patterns to breast milk feeding. In the meantime, concentrations devoid of undesirable effects should be established, since cytotoxicity of biogenic amines has been reported (Reference Linares, del Rio and Redruello87,Reference Del Rio, Redruello and Linares88) .
The present results may have some limitations in inferring to populations since the sample was neither selected randomly nor the evolution over time applies to the same subjects. These were independent samples, which may have introduced some biases. In any case, it should be noted that participants were recruited consecutively (following the inclusion criteria) through the public health system, which serves most of the population in the corresponding geographical area, regardless of their socio-economic and cultural differences.
Acknowledgements
The authors highly appreciate the generosity of the families providing us with the samples used in the study.
This work was supported by Grants from the Universidad de Oviedo (SV-UNOV-10-MA-2, UNO-11-MA-13), Spain.
All authors participated in the design of the study and different aspects to carry it out, mainly L. S., M. M. L. and M. S. analysed the data, and M. S. wrote the article.
There are no conflicts of interest.
Supplementary material
For supplementary material/s referred to in this article, please visit https://doi.org/10.1017/S0007114519001879