Introduction
During breeding season, seabirds are central place foragers and are arguably at greatest risk of suffering from environmental stress due to the combined effects of reduced prey availability from over-fishing (Crawford et al. Reference Crawford, Altwegg, Barham, Barham, Durant, Dyer, Geldenhuys, Makhado, Pichegru, Ryan, Underhill, Upfold, Visagie, Waller and Whittington2011, Grémillet et al. Reference Grémillet, Ponchon, Paleczny, Palomares, Karpouzi and Pauly2018), as well as alterations in breeding habitat (Crawford et al. Reference Crawford, Williams, Hofmeyr, Klages, Randall, Cooper, Dyer and Chesselet1995). In addition, because of their dual reliance on oceans for food and land for breeding, the breeding season is when seabirds would be at their most vulnerable to the effects of climate change as they would have to deal with climate change-related impacts at sea and on land (Sydeman et al. Reference Sydeman, Thompson and Kitaysky2012). In this regard, indirect effects of climate change may have opposite impacts on species, for example, benefitting some species requiring ice-free land to breed (LaRue et al. Reference LaRue, Ainley, Swanson, Dugger, Lyver, Barton and Ballard2013), but negatively impacting those relying on sea ice cover for prey availability (Trathan et al. Reference Trathan, Wienecke, Barbraud, Jenouvrier, Kooyman, Le Bohec, Ainley, Ancel, Zitterbart, Chown, LaRue, Cristofari, Younger, Clucas, Bost, Brown, Gillett and Fretwell2020). However, in general, the direct effects of climate change, such as increasing temperatures and extreme weather events, pose a major threat to the survival of seabirds. In particular, warmer climate seabirds have a high risk of severe hyperthermia and mortality on land with increasing air temperatures (Oswald and Arnold Reference Oswald and Arnold2012), especially those like penguins that have developed adaptations to cold waters (Holt and Boersma Reference Holt and Boersma2022).
Penguins evolved from an ancestral species in the subantarctic regions of Australia–New Zealand and the Antarctic Peninsula (Bertelli and Giannini Reference Bertelli and Giannini2005). They developed efficient morphological and physiological mechanisms to reduce heat loss due to the low temperatures that penguins experienced in water and on land (Williams Reference Williams1995, Luna-Jorquera Reference Luna-Jorquera1996, Dawson and Whittow Reference Dawson, Whittow and Whittow2000), which makes them prone to heat stress. For low-latitude Spheniscus Penguins, adaptations for tolerating cold conditions can be disadvantageous when breeding in hot terrestrial habitats (Luna-Jorquera Reference Luna-Jorquera1996), especially when breeding at exposed sites. As an adaptation, Spheniscus Penguins breed in burrows dug in guano providing a buffered microclimate, with high relative humidity (RH) and little exposure to the wind, as well as protection from aerial predators (Frost et al. Reference Frost, Siegfried and Burger1976). However, due to earlier exploitation by humans of large deposits of guano, most of these penguins are now forced to nest on the surface or under limited vegetation cover, exposing them to the heat of the day (Lei et al. Reference Lei, Green and Pichegru2014). This high level of exposure is detrimental to their populations, as breeding adults tend to leave their nests unattended to go into the sea to cool down and avoid lethal hyperthermia (Frost et al. Reference Frost, Siegfried and Burger1976), which is mostly fatal for the broods because of egg predation and eggs overheating (Yorio and Boersma Reference Yorio and Boersma1994).
Penguin chicks are also vulnerable to heat stress with, for example, downy chicks of Adélie Penguins at risk of lethal hyperthermia if exposed to 7–10°C for a few hours (Chappell et al. Reference Chappell, Morgan and Bucher1990). By contrast, chicks of African Penguins are more adapted to warm temperatures and are known to survive regular exposure to 27–30°C for a few hours (Lei et al. Reference Lei, Green and Pichegru2014). However, given the expected increase in average global temperatures and the increase in extreme heat-wave events due to climate change-related global warming (IPCC 2021), African Penguins are likely to become severely heat stressed in the not-to-distant future. This is of particular concern given their small distribution and greatly reduced population size compared with the start of the 21st century (Crawford et al. Reference Crawford, Altwegg, Barham, Barham, Durant, Dyer, Geldenhuys, Makhado, Pichegru, Ryan, Underhill, Upfold, Visagie, Waller and Whittington2011). The African Penguin is endemic to South Africa and Namibia and following the loss of >70% of its population since 2004 (Crawford et al. Reference Crawford, Altwegg, Barham, Barham, Durant, Dyer, Geldenhuys, Makhado, Pichegru, Ryan, Underhill, Upfold, Visagie, Waller and Whittington2011), it was classified as “Endangered” by the International Union for Conservation of Nature (IUCN) in 2010. Shifts in prey availability and oil spills are major challenges faced by African Penguins while at sea, whereas a reduction in suitable area available to nest in is the major challenge on land due to habitat loss because of former guano scraping (Crawford et al. Reference Crawford, Williams, Hofmeyr, Klages, Randall, Cooper, Dyer and Chesselet1995).
As a conservation effort, artificial housing units have been installed at various African Penguin breeding sites (Kemper et al. Reference Kemper, Underhill, Roux and Kirkman2007, Sherley et al. Reference Sherley, Barham, Barham, Leshoro and Underhill2012, Pichegru Reference Pichegru2013). These artificial housing units, hereafter nest boxes, provide shelter from predators and buffer climate variability, and have proven to be a valuable conservation measure for seabirds (Bolton et al. Reference Bolton, Medeiros, Hothersall and Campos2004, Carlile et al. Reference Carlile, Priddel and Madeiros2012, Libois et al. Reference Libois, Gimenez, Oro, Mínguez, Pradel and Sanz-Aguilar2012) and many other animals (see Beyer and Goldingay Reference Beyer and Goldingay2006, Goldingay and Stevens Reference Goldingay and Stevens2009, Lambrechts et al. Reference Lambrechts, Wiebe, Sunde, Solonen, Sergio, Roulin, Møller, López, Fargallo, Exo, Dell’omo, Costantini, Charter, Butler, Bortolotti, Arlettaz and Korpimäki2012, Rueegger Reference Rueegger2016). Although nest boxes are not without problems related to design, placement, and parasites (Møller Reference Møller1989, Butler et al. Reference Butler, Whitman and Dufty2009, Lambrechts et al. Reference Lambrechts, Wiebe, Sunde, Solonen, Sergio, Roulin, Møller, López, Fargallo, Exo, Dell’omo, Costantini, Charter, Butler, Bortolotti, Arlettaz and Korpimäki2012, Espinaze et al. Reference Espinaze, Hui, Waller and Matthee2020) for example, they are generally an effective tool to facilitate a population recovery. Nest boxes were first installed on Bird Island, Algoa Bay, Eastern Cape, South Africa (33°50’S, 26°17’E) in 2009 to promote population growth in African Penguins (refer to Pichegru Reference Pichegru2013 for full details). To date, several designs of nest boxes have been installed at breeding sites, yielding variable success (Pichegru Reference Pichegru2013, Lei et al. Reference Lei, Green and Pichegru2014). It was found that some of the nest boxes trapped heat, causing prolonged high daily nest temperatures (Lei et al. Reference Lei, Green and Pichegru2014). Subsequently, most of these nest boxes have been removed and a newer design of nest boxes has been installed on Bird Island since 2018, but there has been no monitoring of their success. Therefore, we aimed to compare the microclimate associated with different nests occupied by African Penguins, as well as the birds’ behaviour in response to these temperatures, to determine which type was most effective in buffering variability in temperature and humidity, and so providing the best protection against heat stress and reducing the need to abandon nests.
Methods
Study site
This study took place on Bird Island between 26 February 2021 and 12 March 2021. Bird Island and its surrounding areas in Algoa Bay host nearly half of the global population of African Penguins (Crawford et al. Reference Crawford, Altwegg, Barham, Barham, Durant, Dyer, Geldenhuys, Makhado, Pichegru, Ryan, Underhill, Upfold, Visagie, Waller and Whittington2011). The island has a flat topography with short vegetation (predominantly Tetragonia spp.) and no large trees or boulders to provide shade, resulting in a high level of sun exposure. African Penguins can breed throughout the year (Wolfaardt et al. Reference Wolfaardt, Underhill and Visagie2009), but peak breeding season at the study site is from January to August (Hockey et al. Reference Hockey, Dean and Ryan2005). Although most of the guano has already been removed from the island, some, though very few, natural burrows in guano are present and represent approximately 1% of all breeding nests (Lei et al. Reference Lei, Green and Pichegru2014).
Nest box design
A detailed description of the design and specifications of cement nest boxes can be found in Pichegru (Reference Pichegru2013). Briefly, these nest box designs comprise a 2-cm thick semicircle cement pipe approximately 70 cm (l) × 30 cm (w) × 30 cm (h). The newer nest boxes differ markedly from previous designs and are based on an original description of a natural burrow by Frost et al. (Reference Frost, Siegfried and Burger1976). They are double layered with a 5-cm air gap, have a slanted roof with a 27° angle from the horizontal, the height at the entrance is 20 cm, and the height at the highest point is 50 cm. The length of these nest boxes is 60 cm and their width is 40 cm. They are closed at the back wall, with four holes for ventilation. These nest boxes are hand manufactured by applying several layers of slurry-soaked geotextile over a mould. The slurry consists of a ceramic powder, water, and a waterproofing agent.
Nest microclimates
Owing to the flat topography of the island and the absence of large trees or boulders to provide shade, most of the nests were exposed to similar amounts of direct sunlight, but some penguins do nest in the small shady patches provided by the buildings on the island. We installed temperature and humidity sensitive data loggers (DS1923-F5, Hygrochron iButton, Maxim Integrated, Dublin, Ireland) in 18 nests across the island to record the microclimate of different nest types for comparison. We randomly selected six natural nests including three burrows in guano, two nests under the shade of short natural vegetation cover, and one surface nest in the shade of a building respectively, six half-pipe cement artificial nests, and six double-layered artificial nests. Two data loggers were also installed at other unshaded locations on the island to serve as a measure of the ambient conditions and what penguins in exposed surface nests would experience.
All data loggers were mounted on to a small square (4 × 4 cm) wooden platform to minimise the potential of the recorded temperatures being biased by heat gained through conduction due to contact with the walls of the nests. Data loggers were fixed to the back wall on the inside of the double-layered nests and to the top of the cement nests using a non-hazardous quick drying adhesive (Pattex No More Nails, Henkel Ltd, Durban, South Africa). All data loggers were factory calibrated, where calibrations were made individually in a National Institute of Standards and Technology (NIST)-traceable chamber. However, the calibration of each logger was checked before installation, using a water bath and a precision mercury-in-glass thermometer (resolution = 0.5°C) across a range of temperatures from 4°C to 40°C. The loggers were programmed to record temperature and RH at 10-minute intervals. All but one data logger that was placed in a natural nest were recovered.
Automated cameras (Solar-Dark, Spypoint, Victoriaville, Quebec, Canada) were installed at the entrance of five nests also deployed with iButtons to monitor nest occupancy and penguin behaviour during the hottest period of the day, between 12h00 and 14h00. These included a natural burrow, a cement burrow, and a double-layered nest, as well as one surface nest in a shaded area and one in a sun-exposed area. As the cameras are motion sensitive, we regarded the nest as being occupied on days when images were captured and assumed that it was unoccupied on days when no images were captured. We used these images to estimate crudely the temperatures marking the onset of heat stress in penguins by determining the nest temperature corresponding to images where penguins were visibly heat stressed. To do so, we inspected each image and separated them into one of three groups depending on whether the birds’ beaks were visible or not, and if visible, were the birds panting (head upward, beak gaping) or not. Thereafter, we matched the corresponding nest temperature to the images. For the open and sun-exposed nest, we matched the images to the nearest ambient temperature logger, which was within 5 m of the nest. Of the five cameras installed, the camera monitoring the natural burrow stopped recording images after four days of deployment, suggesting that the burrow was abandoned. Thus, we excluded this camera from all analyses.
Statistical analyses
Following Lei et al. (Reference Lei, Green and Pichegru2014), we estimated maximum daily temperatures, the time at which maximum temperatures occurred, the daily temperature range (difference between minimum and maximum temperature experienced within 24 hours), and the length of time that temperatures exceeded 30°C per day, for each nest type as well as the ambient conditions. Similarly, we also estimated the minimum daily RH levels, the time at which minimum humidity occurred, and the daily RH range (difference between minimum and maximum RH experienced within 24 hours). All but one data logger (natural nest) were retrieved and all but two nests (two natural nests) remained occupied by penguins throughout the study. Therefore, we assumed that penguin occupancy would have a similar influence across nest type and did not warrant further consideration. Our primary interest was the effect of nest type but we included date and nest ID to account for some of the variability in the microclimate data. Comparisons were made using general linear models (GLMs) with nest ID nested within nest type, and nest type and date included as fixed factors. Significant main effects were followed by a Tukey’s honestly significant difference (HSD) post hoc test to determine specific differences between nest types.
We used simple descriptive statistics to analyse camera images, including a histogram plot of the number of images of panting birds versus non-panting birds at various nest temperatures in different nest types.
All statistical procedures were performed using Minitab® Statistical Software and tests were conducted using α = 0.05. Data are presented as the marginal estimated mean ± standard error of the mean (SEM) from the GLM analyses. All experimental protocols were approved by the Nelson Mandela University Animal Ethics Committee (A20-SCI-ZOO-009) and permission to work on Bird Island was granted by the South African National Parks Board.
Results
GLMs showed that there was a significant difference in maximum daily temperature (F3,234 = 279.9, p <0.001) and the daily temperature range (F3,234 = 126.0, p <0.001) between nest types (Figure 1A and B). The post hoc test determined that mean daily maximum temperatures were significantly higher in cement nests (34.3 ± 0.8°C) than in any other nest types, whereas those in double-layered ceramic nests (27.5 ± 0.3°C) were significantly lower than all the others. There was no difference between natural shaded nests and exposed surface nests (mean daily maximum temperatures: 29.3 ± 0.8°C versus 29.8 ± 1.1°C, respectively). Similarly, the daily temperature range was largest in cement nests (16.7 ± 0.8°C), similar between natural shaded nests (11.2 ± 0.9°C) and exposed nests (12.4 ± 1.1°C), and smallest in double-layered ceramic nests (7.8 ± 0.3°C).
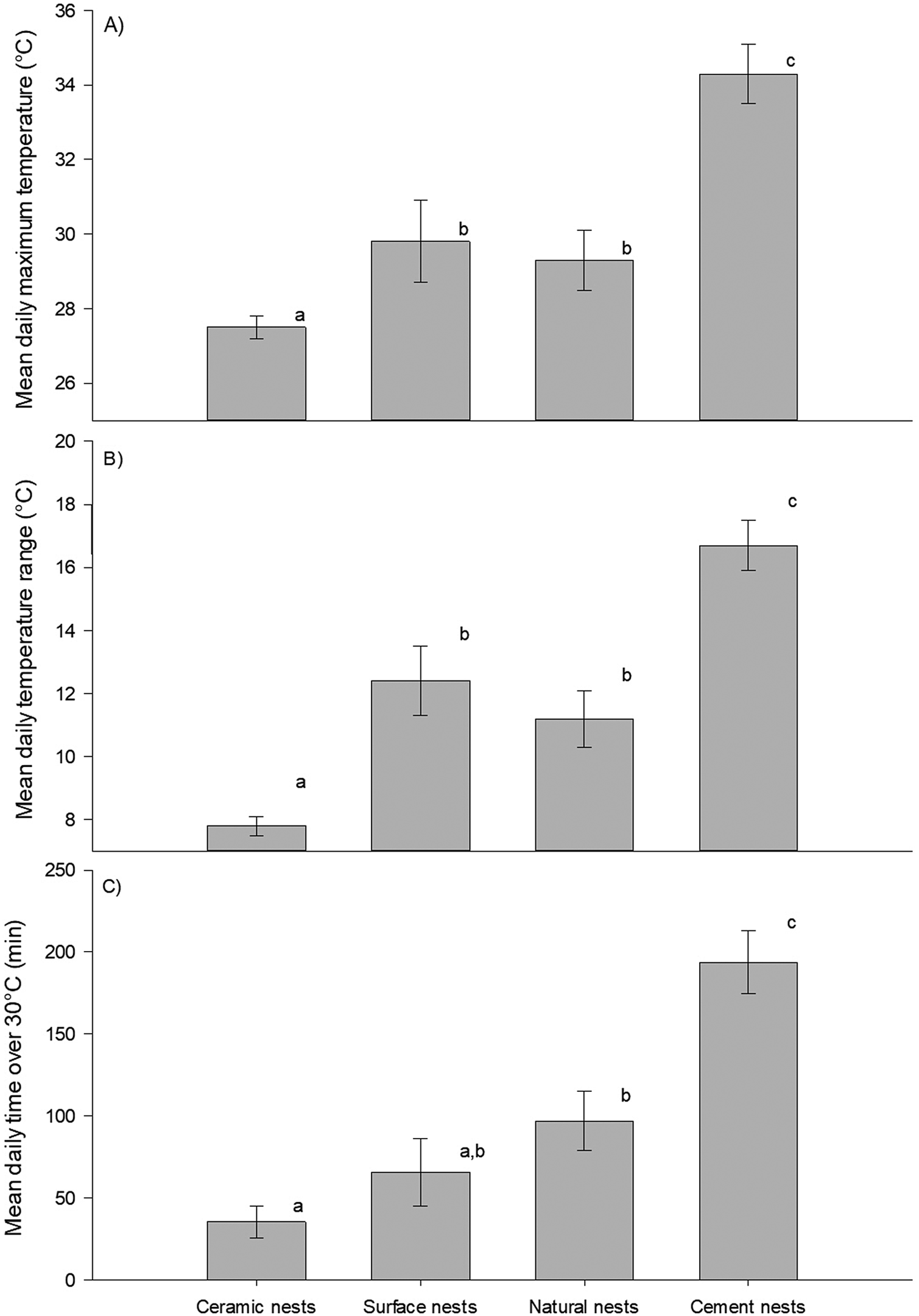
Figure 1. Daily temperatures in African Penguin (Spheniscus demersus) nests on Bird Island, Algoa Bay, South Africa between 26 February 2021 and 12 March 2021. (A) Daily maximum temperatures; (B) daily temperature range; (C) length of time per day during which temperature exceeds 30°C. Dissimilar letters in each plot indicate significant differences.
Importantly, the length of time with temperatures above 30°C during our study differed significantly between nest types (F3,234 = 44.7, p <0.001). Over the 14 days of our data collection, temperatures exceeded 30°C, on average, for approximately 3 hours 14 minutes per day in cement nests, 1 hour 37 minutes in natural shaded nests, 1 hour 6 minutes in exposed surface nests, and only 35 minutes in double-layered ceramic nests. Post hoc tests revealed that cement nests were hot for longer than any other nests, while the length of time spent above 30°C was similar between exposed surface nests and natural shaded nests, as well as between double-layered ceramic nests and exposed surface nests (Figure 1C). Extreme temperatures of over 40°C were observed twice in one of the ambient temperature loggers, on six occasions in one of the natural burrows, and on 16 occasions across four of the cement nests, with maximum temperatures even exceeding 50°C on two of those occasions (in the same cement nest). By contrast, the absolute highest temperature recorded within any of the double-layered ceramic nest boxes was 36.7°C.
There was a significant difference in the time at which maximum temperatures were observed between nest types (F3,234 = 3.93, p <0.05). The post host test determined that the time at which temperatures peaked differed between cement nests and natural shaded nests, but no other differences were found. Temperatures peaked in cement nests at approximately 12h54 and a little over an hour later in natural shaded nests at approximately 14h00. Temperature peaked in exposed surface nests at approximately 13h02 and at approximately 13h21 in double-layered ceramic nests (Figure 2A).
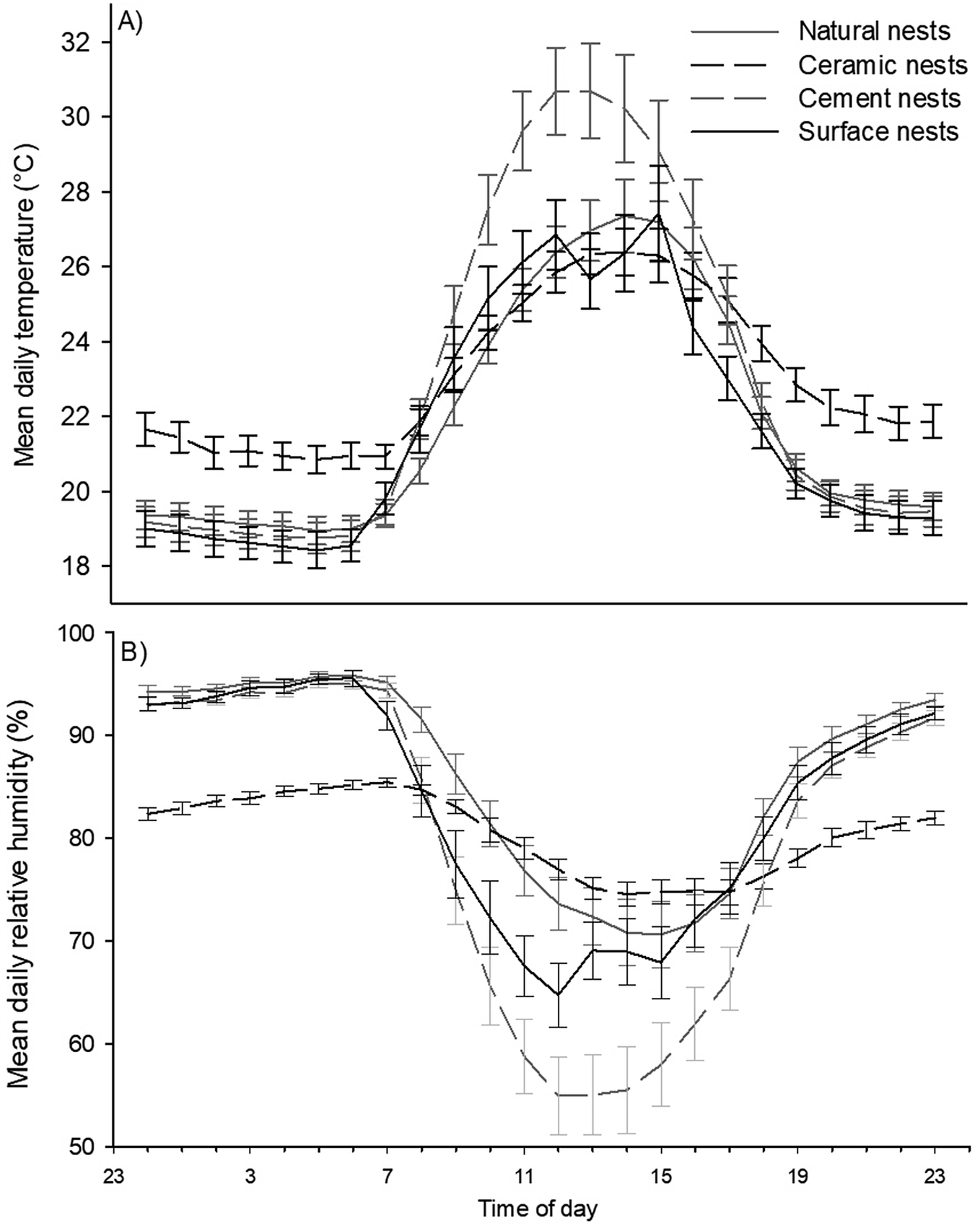
Figure 2. Hourly temperatures (A) and humidity levels (B) in African Penguin (Spheniscus demersus) nests on Bird Island, Algoa Bay, South Africa between 26 February and 12 March 2021.
A total of 1,108 images were recorded, of which 80% (N = 888) were exploitable, i.e. the images showed a penguin occupying the nest and its beak could be seen distinctly. Few images were recorded from double-layered ceramic nests (N = 149 compared with N = 339 for natural shaded nests or N = 296 for exposed surface nests) due to the low visibility inside the nest. Of these 888 images, 35% showed birds in heat stress. The lowest temperatures at which heat stress was visible was at 22°C in exposed surface nests, at 25°C in both the shaded natural nests and the double-layered ceramic nest box, and at 29°C in the cement nest (Figures 3 and 4). Overall, there was a change in the proportion of images captured showing birds in heat stress versus not stressed between nest temperatures of 24°C and 25 °C (Figure 4). At nest temperatures ≥25°C there were always more images of heat-stressed birds than not when combining all nest types together, and at nest temperatures ≥36°C there were only images of heat-stressed birds (Figure 4).
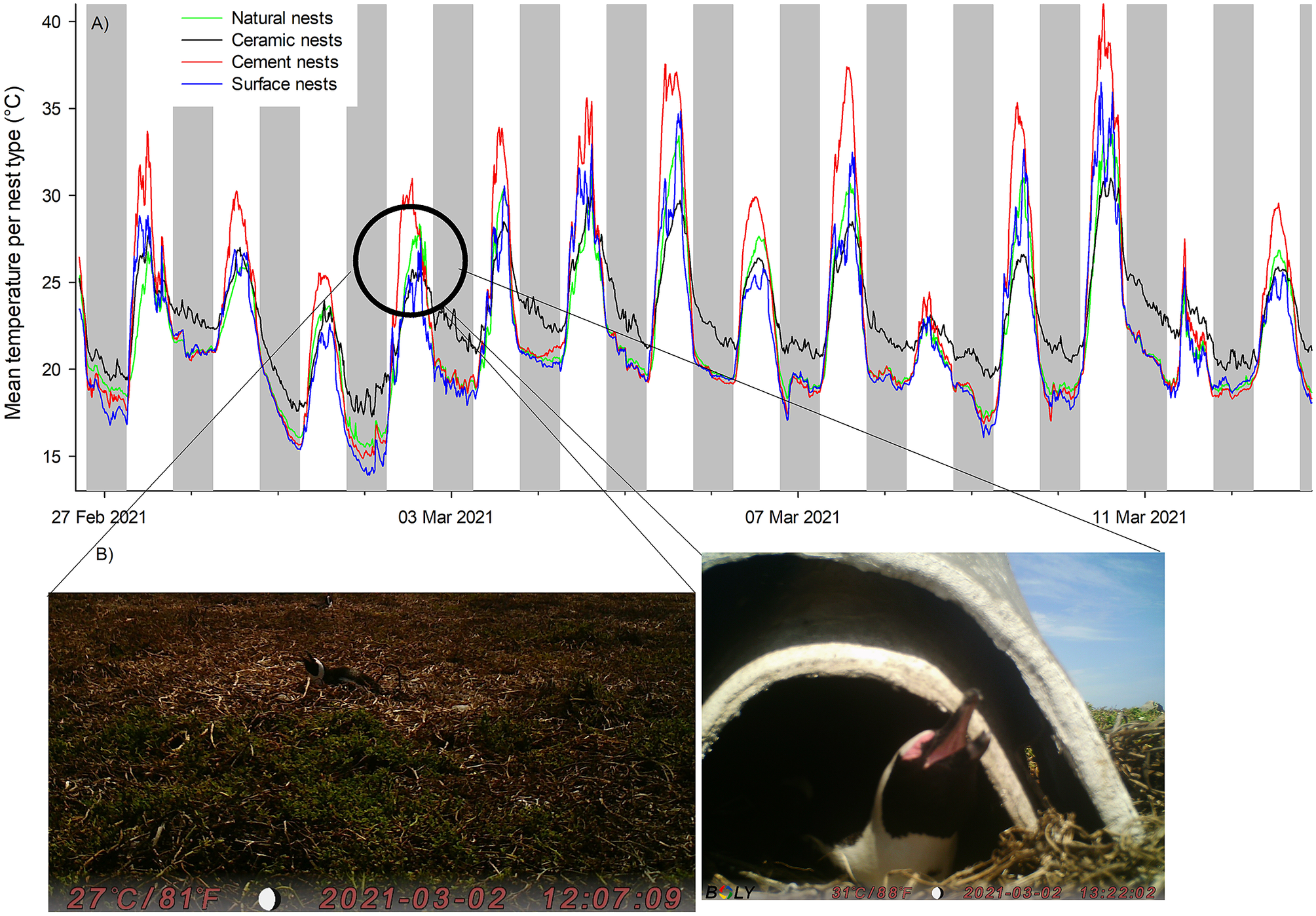
Figure 3. (A) Temperature profile of nests and (B) corresponding images of adult African Penguins (Spheniscus demersus) panting at nest temperatures of approximately 25°C on Bird Island, Algoa Bay, South Africa between 26 February 2021 and 12 March 2021. The image on the left is of a penguin in a surface nest and the image on the right is of a penguin in a double-layered nest.
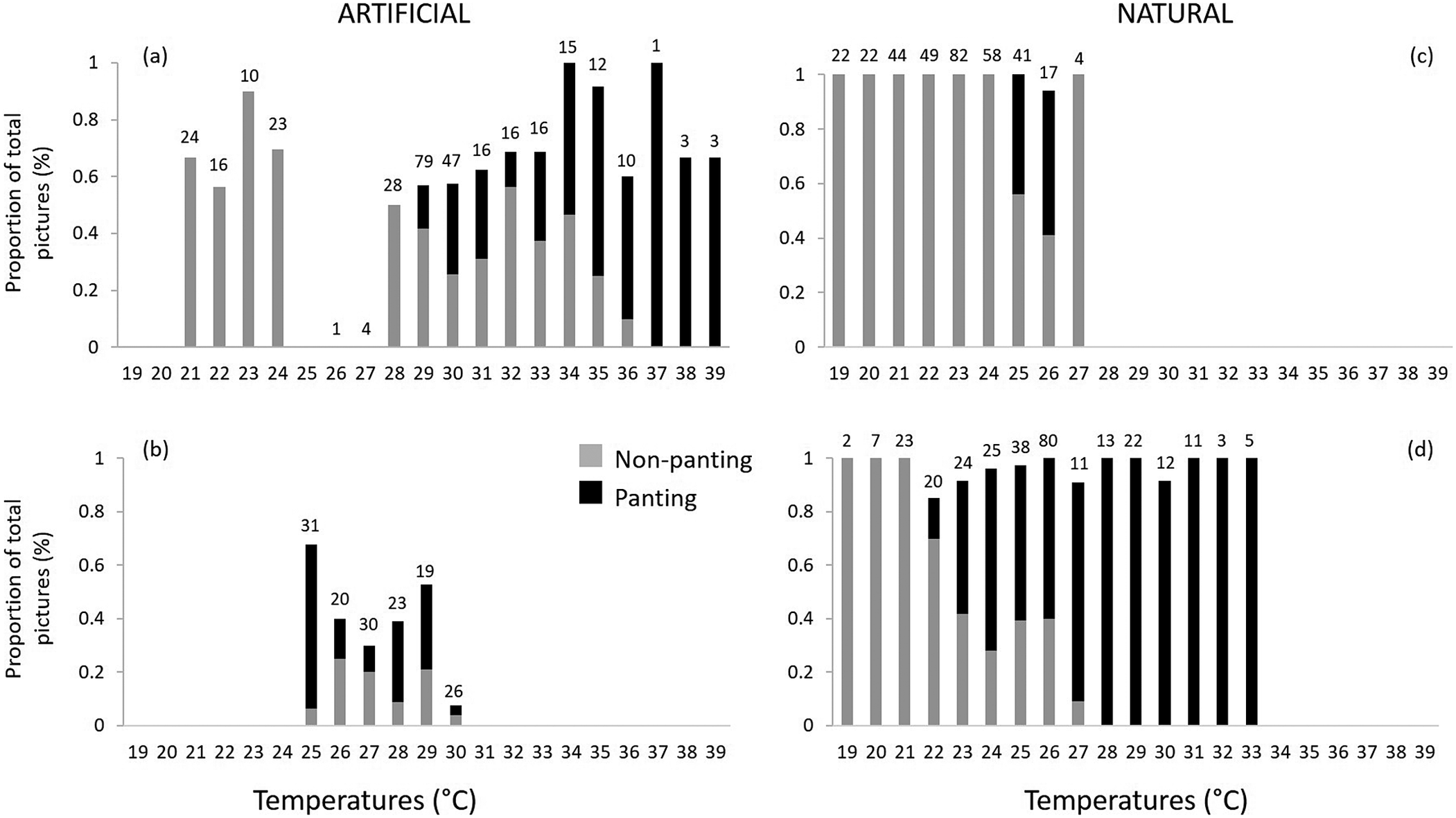
Figure 4. Proportion of images of African Penguins (Spheniscus demersus) visibly heat stressed (panting – in black) and non-panting (light grey) from the total number of images recorded at each temperature (depicted above each bar) in four nest types: artificial (A) cement pipe nests and (B) double-layered ceramic nests; and natural (C) shaded and (D) exposed surface nests. Images were recorded between 12h00 and 14h00 daily on Bird Island, Algoa Bay, South Africa between 26 February 2021 and 12 March 2021.
Finally, mean daily minimum humidity (F3,234 = 102.8, p <0.001) and its range (F3,234 = 179.1, p <0.001) differed significantly between nest types (Figure 5A and B). The post hoc tests showed that mean daily minimum humidity and mean daily humidity range differed between all nest types. Further, the data showed opposite trends for these variables. Cement nests had the lowest daily minimum humidity but the highest humidity range (minimum RH = 48.3 ± 1.7%; range: 48.5 ± 1.7%), followed by the surface nests (minimum RH = 60.0 ± 2.4%; range: 37.6 ± 2.3%), then by natural nests (minimum RH= 65.8 ± 2.6%; range: 31.5 ± 2.5%), and lastly double-layered ceramic nests with the highest minimum daily humidity and smallest range (minimum RH = 70.3 ± 1.0%; range: 18.6 ± 0.8%). The time at which temperature peaked coincided closely with the approximate time at which humidity was lowest namely, at 12h44 in surface nests, 13h15 in cement nests, 13h18 in double-layered ceramic nests, and 14h00 in natural nests (Figure 2B). However, unlike with the time at which temperature peaked, the statistical analysis could not distinguish between these times.
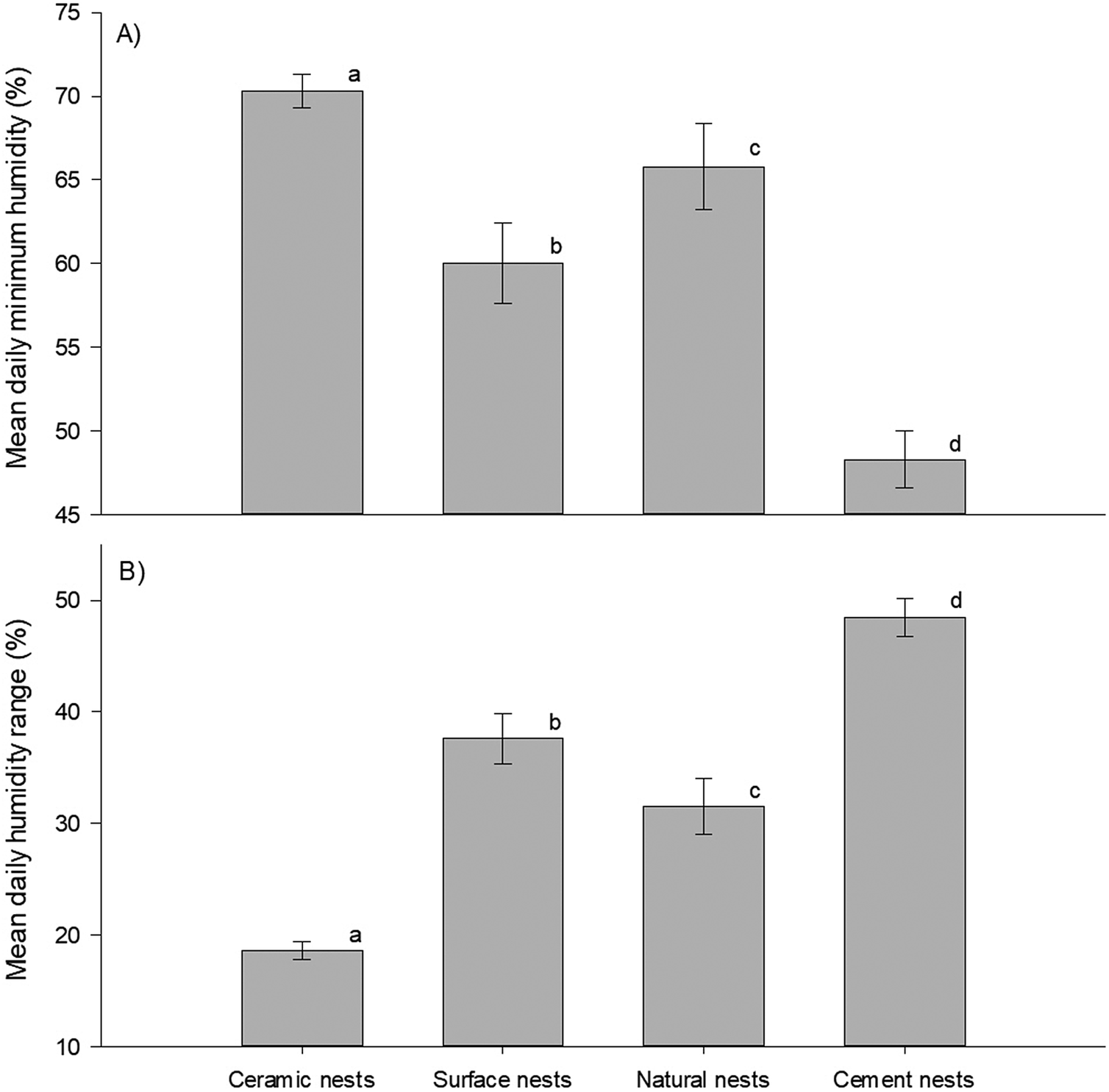
Figure 5. Daily humidity levels in African Penguin (Spheniscus demersus) nests on Bird Island, Algoa Bay, South Africa between 26 February 2021 and 12 March 2021. (A) Daily minimum humidity and (B) daily humidity range. Dissimilar letters in each plot indicate significant differences.
Discussion
In response to the drastic decline in the number of African Penguins globally within the last century, a Biodiversity Management Plan was set in place in 2013, recommending various conservation measures to be implemented to prevent its extinction. As part of this plan, managing authorities intervened by installing artificial nests at various breeding sites to protect penguins from extreme weather events and predation (Pichegru Reference Pichegru2013). Several versions of these artificial nests have been tried with variable success (Kemper et al. Reference Kemper, Underhill, Roux and Kirkman2007; Sherley et al. Reference Sherley, Barham, Barham, Leshoro and Underhill2012; Pichegru Reference Pichegru2013; Espinaze et al. Reference Espinaze, Hui, Waller and Matthee2020) and Lei et al. (Reference Lei, Green and Pichegru2014) recommended the use of cement nests, which at the time showed higher breeding success than surface nests or fibreglass artificial nests in African Penguins breeding on Bird Island. However, these cement nests still posed a threat to penguins because of the high temperatures that they reached (above 50°C on occasions). Until now, nest boxes on Bird Island have only been effective at preventing egg predation by gulls but have provided little protection against heat. This was concerning as latest predictions by the Intergovernmental Panel on Climate Change (IPCC) suggest a significant increase in the frequency and intensity of extreme weather events, even under the lowest greenhouse gas emission scenario, a four-fold increase in extreme heat events is expected (IPCC 2021). Here, we show that the newest edition of nest boxes, with a 5-cm layer of air trapped between two layers of material for insulation, was highly effective at buffering environmental variability, even compared with natural burrows and shaded nests.
Mean daily humidity in double-layered ceramic nests was also higher and more stable compared with all other nests, and mean daily maximum temperature was lower, with less variability throughout the day. As our study coincided with the mid-to-late incubation phase for the penguins at Bird Island, the results have a direct bearing on hatching success and fledgling survival. While we could not assess breeding success and compare it between nest types due to logistical constraints, we expect a higher success rate in double-layered ceramic nests because they provide similar protection against predation as cement nests and natural burrows, but significantly better protection against overheating. The absolute maximum temperature observed within these new nests was approximately 1°C lower than the resting body temperature of African Penguins (37.3°C) (Wilson and Grémillet Reference Wilson and Grémillet1996). This means that if left unattended when the incubating adult leaves its nest to cool down at sea, penguin eggs in these nests were never at risk of overheating. In other nests, if adult penguins were to neglect eggs to cool off by swimming in the sea (Frost et al. Reference Frost, Siegfried and Burger1976), overheating in eggs was a possibility because temperatures exceeded 40°C on occasion, even amongst natural burrows.
An argument could be made that differences in the placement of data loggers in the various nest types may have biased the temperatures recorded in our study. For example, because cement pipes are lower and nests were occupied by incubating penguins, the heat emitted by the birds may have elevated the temperatures recorded in cement nests compared with those of other nests where the data loggers were further away from the birds. While we cannot rule out this possibility, penguins evolved effective mechanisms to retain body heat in frigid conditions and thus radiative heat transfer through their feathers is low (Dawson et al. Reference Dawson, Vincent, Jeronimidis, Rice and Forshaw1999), and they do not have large thermal windows available to dissipate excess body heat (McCafferty et al. Reference McCafferty, Gilbert, Thierry, Currie, Le Maho and Ancel2013). Instead, to offload excess body heat while on land they would rely on evaporative cooling through panting, with the water vapour being at a similar or lower temperature than their body temperature. Based on this, it is unlikely that penguins would have had any meaningful contribution to temperatures recorded. Thus, we argue that, at the very least, the extreme nest temperatures of 40°C or more recorded in this study (i.e. above body temperature) were a true reflection of the temperatures experienced at the time. As such, the lower temperatures in the new nest type are encouraging because our results also suggest that African Penguins may be less tolerant of heat than previously thought.
By integrating the images of penguins from cameras stationed at nests around the island with that of nest-specific microclimatic data, we provide evidence that heat stress in African Penguins may start at temperatures that are 5°C lower than in Humboldt Penguins, a species which has been used as a benchmark for thermotolerance in the past (Lei et al. Reference Lei, Green and Pichegru2014). Our estimation of the temperature marking the onset of heat stress was based on the stark increase in the number of sightings of penguins panting at 25°C, including penguins in shaded nests, compared with sightings at lower temperatures. Importantly, observations of heat stress in penguins making use of exposed surface nests were made at 22°C already (Figures 3 and 4). The apparent heat stress at a lower ambient temperature in surface nests is to be expected as penguins in exposed nests would also have to combat a large amount of exogenous passive heat gained from direct solar radiation (Tattersall et al. Reference Tattersall, Sinclair, Withers, Fields, Seebacher, Cooper and Maloney2012). Worryingly, a large proportion of penguin nests on Bird Island are exposed and thus susceptible to severe heat stress in the not-too-distant future due to global warming.
We recognised that our estimation of heat stress was likely confounded by the difficulty in obtaining a constant and clear view of penguins’ faces and because we did not quantify the heat-exchange dynamics between the birds and their surroundings. With regards to the first problem, remote camera traps are likely to underestimate the frequency of occurrence of panting within artificial nests, compared with surface nests. This is especially true for ceramic nests, where penguins would not be seen inside. Thus, data for these and other non-surface nest types were biased towards recording panting events when the birds were coming outside in clear view of the camera. With regards to the issue of not quantifying the heat-exchange dynamics between birds and their surroundings, it is likely that our interpretation of the temperatures stressful to penguins were biased towards slightly higher temperatures on windy days. This is because penguins may better cope with high ambient temperatures on windy days due to enhanced convective cooling, provided that there is sufficient air flow through the nest. For example, the open-ended design of cement nests may have promoted convective cooling, whereas the small openings in the back walls of ceramic nests may not. Together with the challenges of obtaining a clear view of penguins in nests, enhanced convective cooling could explain why there were no observations of panting in penguins in cement nests at temperatures below 29°C. However, we caution that this should not be misinterpreted as penguins faring better in cement nests, because temperatures in these nests were typically much higher than in other nests and remained above 30°C for much longer. This is likely because cement absorbs and retains much of the solar heat, and unlike ceramic nests, it is a solid block without an air gap to act as insulation by trapping some of the heat.
Given the limitations of our study, it is crucial that our estimation of the onset of heat stress be validated by controlled thermoregulatory studies that can quantify the total heat burden faced by penguins under different scenarios. A further benefit of such thermoregulatory studies would be that they allow for more accurate estimation of energy expenditure associated with thermoregulation. Based on a threshold of 30°C, it was argued that African Penguins on Bird Island nesting in artificial nests may have increased energy expenditure associated with thermoregulation for up to 16 times longer in a day than those in natural burrows (Lei et al. Reference Lei, Green and Pichegru2014). Following the same approach, the results suggested that ceramic nests provide a significant energy conservation benefit. Double-layered ceramic nests have much lower thermoregulatory costs compared with cement nests as the double-layered ceramic nests spent only a small fraction (
$ \frac{1}{5} $
th) of time above 30°C compared with cement nests. However, these predictions do not consider the potential influence that convective cooling may have on energy expenditure. By contrast, given that ceramic nests were designed based on natural burrows, with similar levels of airflow, penguins using the newer double-layered nest type may actually have lower thermoregulatory costs compared with natural burrows.
In concert with nest temperature, moisture is also important for successful breeding and Lei et al. (Reference Lei, Green and Pichegru2014) observed mean hourly humidity levels in cement nests that were always above 60% and mean daily minimum humidity levels of c. 55%. In our study, mean hourly humidity values in cement nests routinely decreased to less than 60% between 11h00 and 15h00, coincident with midday heat. Also, we observed mean daily minimum humidity levels that were 10% lower than the previous account. This poses a serious risk of eggs losing too much moisture. During incubation, African Penguin eggs typical lose 13–15% of their mass through evaporation (Klusener et al. Reference Klusener, Hurtado, Parsons, Vanstreels, Stander, Van Der Spuy and Ludynia2018). The Southern African Foundation for the Conservation of Coastal Birds (SANCCOB), who developed a successful egg incubation and hand-rearing programme to assist with the population recovery of African Penguins, recommended that eggs initially be incubated at 36.5°C and 40% humidity and later, once eggs externally pitted, humidity be increased to 60%. The increased humidity was necessary to prevent the egg membranes from desiccating (Klusener et al. Reference Klusener, Hurtado, Parsons, Vanstreels, Stander, Van Der Spuy and Ludynia2018). Therefore, current observations showed that eggs in cement nests may have faced water stress in addition to heat stress, whereas the risk of this was minimal in the newer nest type.
In the past, cement nests have been shown to positively influence breeding success, despite the challenges with extreme temperatures on hot days (Lei et al. Reference Lei, Green and Pichegru2014). Presumably, humidity levels were adequate and the fact that cement nests are open on both ends allowed for sufficient cooling to prevent lethal hyperthermia in nesting penguins and their eggs. Looking ahead, based on the results of the current study, it seems likely that as environmental conditions become hotter, with more extreme weather events due to global warming, cement nests will become an ineffective conservation tool. Fortunately, the double-layered ceramic nests performed as well as, if not better than, natural burrows, providing ideal conditions for penguins to breed. Based only on microclimate, we advocate that these nests are appropriate to improve breeding conditions on land for African Penguins. However, we recommend that future work consider the orientation of nests relative to the direction of the prevailing winds at breeding sites and compare the airflow within each nest type. This would improve the general understanding of the contribution of convective cooling in preventing heat stress in chicks and adult penguins, and how it may increase the risk of desiccation in eggs. Future studies should also consider the potential impact of parasites. For example, even though ceramic nests may provide an ideal microclimate for breeding, parasites may build up in the nests, forcing the birds to move breeding sites (sensu Espinaze et al. Reference Espinaze, Hui, Waller and Matthee2020), resulting in a decline in usage of these nest types in future. This type of approach would facilitate a more effective site-specific management plan, especially when combined with data for breeding success.