Introduction
It is commonly understood that drilling is a process of creating a borehole, and one of the main technological elements of drilling, including access holes in subglacial environments, is the removal of borehole products when cutting or melting the ice. The removal of material is critically important to all drilling systems, as the presence of excessive material at the bottom of the borehole leads to decreasing penetration rates and even loss of the drill. For intermediate and deep drilling, it is also necessary to prevent borehole closure through visco-plastic deformation by filling the borehole with a fluid (Reference Talalay and HookeTalalay and Hooke, 2007).
Review of the properties of fluids used for drilling in permafrost confirms that they are not suitable for drilling in cold ice (Reference Chistyakov, Talalay, Yakovlev and YakovlevChistyakov and others, 1999; Reference TalalayTalalay, 2011), which is why various one- or two-compound low-temperature drilling fluids were utilized under these circumstances (Reference Talalay and GundestrupTalalay and Gundestrup, 2002; Reference GerasimoffGerasimoff, 2003). Three types of borehole fluid have been used in ice-core drilling: (1) two-component kerosene-based fluids with density additives, (2) alcohol compounds and (3) ester compounds.
Water is also used as a circulation medium in hot-water drilling systems. In this case, water has two main functions: (1) to convey heat to the bottom of the hole to melt the ice and (2) to remove melted water by mixing with pumped hot water. The main advantages of exploration of the subglacial environment with hot-water drilling systems are that the equipment can provide cleaner samples of subglacial water and sediments and that access to the ice-sheet base is rapid. On the other hand, hot water, even when heated to 90°C, filtered to 0.2 m m and ultraviolet (UV)-treated at the surface, could pick up microorganisms from near-surface snow and circulate them in large numbers through the borehole. In addition, in cold ice the long-term radial freeze rate is ~0.4cm h–1, with an initial freeze rate up to four times higher (personal communication from K. Makinson, 2013), which makes subglacial exploration extremely difficult. Another negative impact of the hot-water circulation medium is thermal pollution of glacial ice and subglacial water, which changes the ambient temperature around the borehole. Although penetration into the subglacial environment by hot-water drilling technologies is technically possible, we do not consider water as a ‘low-temperature’ drilling fluid.
Hereinafter we review the main constituents of the special low-temperature drilling fluids with freezing (pour) points lower than the minimal temperature in the borehole. The average annual temperature in central Antarctica (and thus the minimal borehole temperature) at 10 m depth is in the range –508C to –588C (Reference King and TurnerKing and Turner, 1997), and this can be taken as the anti-freezing requirement of low-temperature drilling fluids. The effects of drilling fluids on the natural environment are analyzed from the following points of view: (1) occupational safety and health; (2) ozone depletion and global warming; (3) chemical pollution; and (4) biological pollution.
Drilling Fluid Compositions
Two-component kerosene-based fluids
Two-component kerosene-based fluids are based on kerosene-like products, such as the low-temperature jet fuels Jet A1 and TS-1, or solvents of the EXXSOLTM D-series and IsoparTM K (Table 1). These fluids are all very similar in function but differ in the content of aromatics, waxes, sulfur and other impurities. Kerosenes have a density of ~800-850 kg m–3 at -30°C compared with 917-924 kg m–3 for ice. Therefore, they are made denser by mixing with fluorocarbons or other compounds with a density that significantly exceeds the density of ice (Table 2).
Table 1. Reference data for low-temperature drilling fluid components.
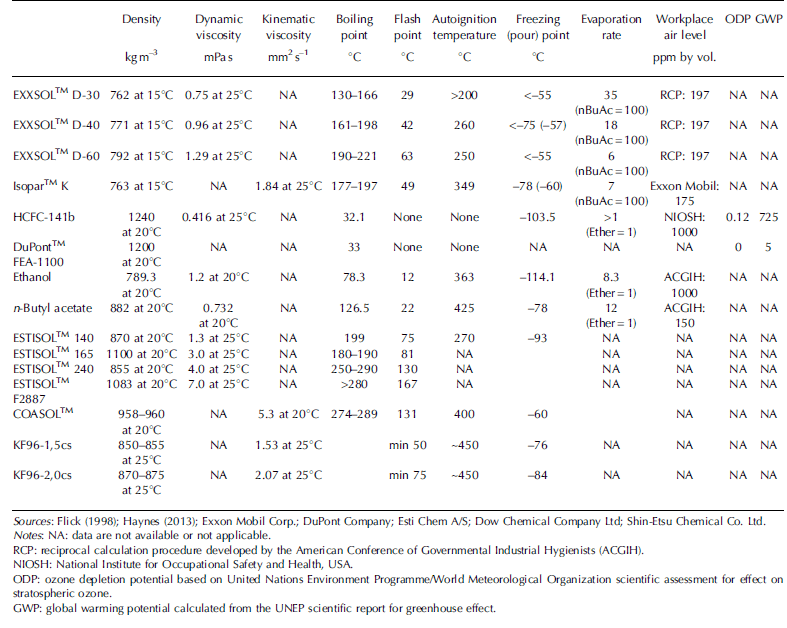
Table 2. Estimation of the main technological properties of low-temperature drilling fluids.

During the past 20 years many of the boreholes drilled in Antarctica and Greenland have been successfully completed using a mixture of a kerosene-like fluid and dichlorofluoro-ethane HCFC-141b as densifier (North Greenland Icecore Project (NGRIP)-2, 3095 m depth; European Project for Ice Coring in Antarctica (EPICA) Dome C2, 3270 m depth; EPICA Dronning Maud Land (DML), 2774 m depth; Berkner Island, 998 m depth; Talos Dome, 1620 m depth; West Antarctic ice sheet (WAIS) Divide, 3405 m depth; Vostok station, 3769 m depth). Recently three boreholes in which drilling fluid of this type was used reached subglacial water, and in all cases subglacial water flushed the drill fluid back into the under-pressurized boreholes (Table 3). As fluorocarbons are known to play a role in ozone depletion, at the present time the production and use of HCFC-141b is banned in some countries and in others has been stopped or is gradually being reduced. Unfortunately, attempts to find another appropriate densifier for two-component kerosene-based fluids have been unsuccessful.
Table 3. Experience of accessing subglacial water with kerosene-based drilling fluids.

The Ice Drilling Design and Operations group (IDDO; University of Wisconsin-Madison, USA) tested a two-compound fluid consisting of IsoparTM K and segregated hydrofluoroether HFE-7100 produced by the 3M Corporation (personal communication from M. Gerasimoff, 2004). Experimental tests showed that this mixture separated into two phases over a very narrow temperature range at ~–45°C; this makes it unusable in boreholes in cold ice.
The Centre for Ice and Climate at the University of Copenhagen, Denmark, investigated as densifier the di-isobutyl-ester of succinyl-, glutar- and adipinacid (2:4:3) produced by the BASF chemical company under the Lusolvan® FBH trademark (personal communication from S. Sheldon, 2011). Lusolvan® FBH is readily miscible with EXXSOLTM D40 solvent. The viscosity of this mixture is very high, which would have an undesirable influence by slowing the tripping operation in the borehole. To achieve a higher free lowering rate the borehole could be drilled with a larger clearance (10-12 mm) between the drill and borehole walls. However, this would lead to a significant increase in the volume of cuttings (or melted water), shorter run duration, decreased ice production rate, increased power consumption and so on.
The Polar Research Center at Jilin University studied two types of densifier: decafluoropentane HFC-4310mee and ESTISOLTM F2887, a synthetic ester made from a polyvalent alcohol produced by Esti Chem A/S. It was found that HFC-4310mee is not miscible with EXXSOLTM D40 solvent at any observed temperatures, and ESTISOLTM F2887 is miscible with EXXSOLTM D40 at room temperature but the mixture separates out into immiscible liquids at subzero temperatures.
In principle, it would be interesting to test as a densifier for kerosene-based drilling fluids a fourth-generation foam-expansion agent DuPontTM FEA-1100, which is a hydrofluoroolefin of the HFO-1336mzz type (Reference Loh, Creazzo and RobinLoh and others, 2009). It has high solubility in organic compounds, but the miscibility with kerosene is questionable. DuPontTM FEA-1100 is characterized by good environmental properties and compatibility with the common metals, plastics and elastomers. Commercial sale of DuPontTM FEA-1100 is planned for 2013, but at the time of writing it is not available on the market.
Thus, at present a new suitable densifier for two-component petroleum-based fluids has not been found. It seems logical to focus future research on the identification of new non-toxic, non-flammable, density-appropriate and environmentally friendly non-petroleum fluids because use of kerosene as the main component in these fluids is hampered by its toxicity.
Alcohol compounds
During the past few decades, many holes in ice were drilled with ethanol–water solution (Reference Zagorodnov, Kelley and NagornovZagorodnov and others, 1994). This is a hydrophilic liquid, which dissolves water and ice up to the equilibrium concentration at a given temperature. Drilling technology involving this kind of fluid is reliant on the removal of ice cuttings or melted water by dissolution. Therefore, the amount of drilling fluid required is less than when drilling with a hydrophobic drilling fluid as the total volume of liquid is 5–75% of borehole volume depending on the temperature profile of fluid in the borehole. The use of ethanol for accessing Vostok Subglacial Lake was discussed at the first stage of project development (Reference Chistyakov and TalalayChistyakov and Talalay, 1998), but the idea was subsequently dismissed because of the strong aseptic properties of ethanol.
Hydrophilic liquids dissolve ice not only at the borehole bottom, but also from the borehole walls. In the case of borehole temperature changes (e.g. due to convection processes in the borehole), the water freezes out from aqueous solution and forms slush in the borehole. The other property that has limited the use of ethanol in aqueous solution is its very high viscosity.
Ester compounds
At the end of the 1980s, specialists from the Polar Ice Coring Office (PICO, University of Alaska Fairbanks, USA) suggested using as a low-temperature drilling fluid n-butyl acetate, an ester used mainly as a solvent in the nitrocellulose and cellulose industries (Reference Gosink, Kelley, Koci, Burton and TumeoGosink and others, 1991, Reference Gosink1994). In the following years, two deep-drilling projects were completed with this fluid, within the Greenland Ice Sheet Project 2 (GISP2), Summit, Greenland (3053 m), and at Dome F, Antarctica (3035 m). At the preliminary stage, n-butyl acetate has been chosen as the drilling fluid for the forthcoming deep ice-core drilling project at Dome A, Antarctica (Reference TalalayTalalay and others, 2014).
n-Butyl acetate has a low initial purchase cost, but is an ongoing liability from a safety (fire and explosion) and acute/chronic health hazard standpoint. The main problem of using n-butyl acetate as a drilling fluid is the hazard it presents to the physical and mental health of the people working at the drilling site. n-Butyl acetate is a very aggressive solvent: there are no elastomers that can operate for a long time in n-butyl acetate.
The new type of ester mixtures produced initially by Dow Chemical Company and since 2006 by Esti Chem A/S under the trademark ESTISOLTM have become the preferred alternatives to aliphatic and aromatic hydrocarbons in formulated chemical products. In industry, ESTISOLTM esters can act as straight replacements, solvency boosters or carrier fluids in formulated products (e.g. industrial cleaners, degreasers, blanket and roller washers, printing inks, hand cleaners, paint strippers and oilfield chemicals).
Danish specialists from the Centre for Ice and Climate chose for laboratory and field-testing a mixture of ESTISOLTM 240 and COASOLTM (Reference Larsen, Sheldon, Steffensen and IceLarsen and others, 2011). COASOLTM is the same mixture of refined di-isobutyl esters as the above-mentioned Lusolvan® FBH. ESTISOLTM 240 and COASOLTM are characterized by low vapor pressure, almost no odor, low toxicity and good biodegradable properties.
Another promising product of Esti Chem A/S is the aliphatic synthetic ester ESTISOLTM 140, a clear fluid with a fruit-like odor, which is immiscible with water. ESTISOLTM 140 is almost sufficiently dense by itself (Fig. 1; Table 4) to compensate for ice overburden pressure, but in some cases, to provide long-term stability of the borehole walls, two other ESTISOLTM products, ESTISOLTM 165 and ESTISOLTM F2887, added in small quantities (2–6 wt%) could be used as densifiers for ESTISOLTM 140-based drilling fluids. Tests showed that ESTISOLTM 140 is miscible with ESTISOLTM 165 and ESTISOLTM F2887, and the density–temperature relationship is linear.

Fig. 1. Density of single- and two-component ESTISOLTM esters vs temperature.
Table 4. Experimental density–temperature equations of the single- and two-component ESTISOLTM esters under atmospheric pressure.

From a simple geometric model in the case when the components do not react either chemically or physically (i.e. each molecule component occupies on average a fixed volume), the density of ESTISOLTM 140-based drilling fluids can be obtained at any desirable concentration according to

for a mixture of ESTISOLTM 140 and ESTISOLTM 165, and

for a mixture of ESTISOLTM 140 and ESTISOLTM F2887, where Fm (kg kg–1) is the mass fraction of densifier (ratio of densifier mass to the mass of the total mixture).
The viscosity of single- and two-compound ESTISOLTM esters was measured using a DC-6506 type rotational viscometer (Fangrui Instrument Co. Ltd) with an embedded thermostat that can maintain the temperature in the range –65°C to 100°C. In contrast to density, viscosity does not remain in direct proportion to temperature: at low temperatures viscosity increased in an exponential relationship (Fig. 2; Table 5). The viscosity of single ESTISOLTM 165 and F2887 esters is much higher than that of ESTISOLTM 140 ester, but adding small amounts of these esters to the base fluid has almost no influence on the viscosity of the two-component mixture. The viscosity of ESTISOLTM 140-based fluids can be considered reasonable at borehole temperatures down to -50°C; at temperatures below -50°C the relatively high viscosity may influence the travel time of the drill and therefore the total drilling time.
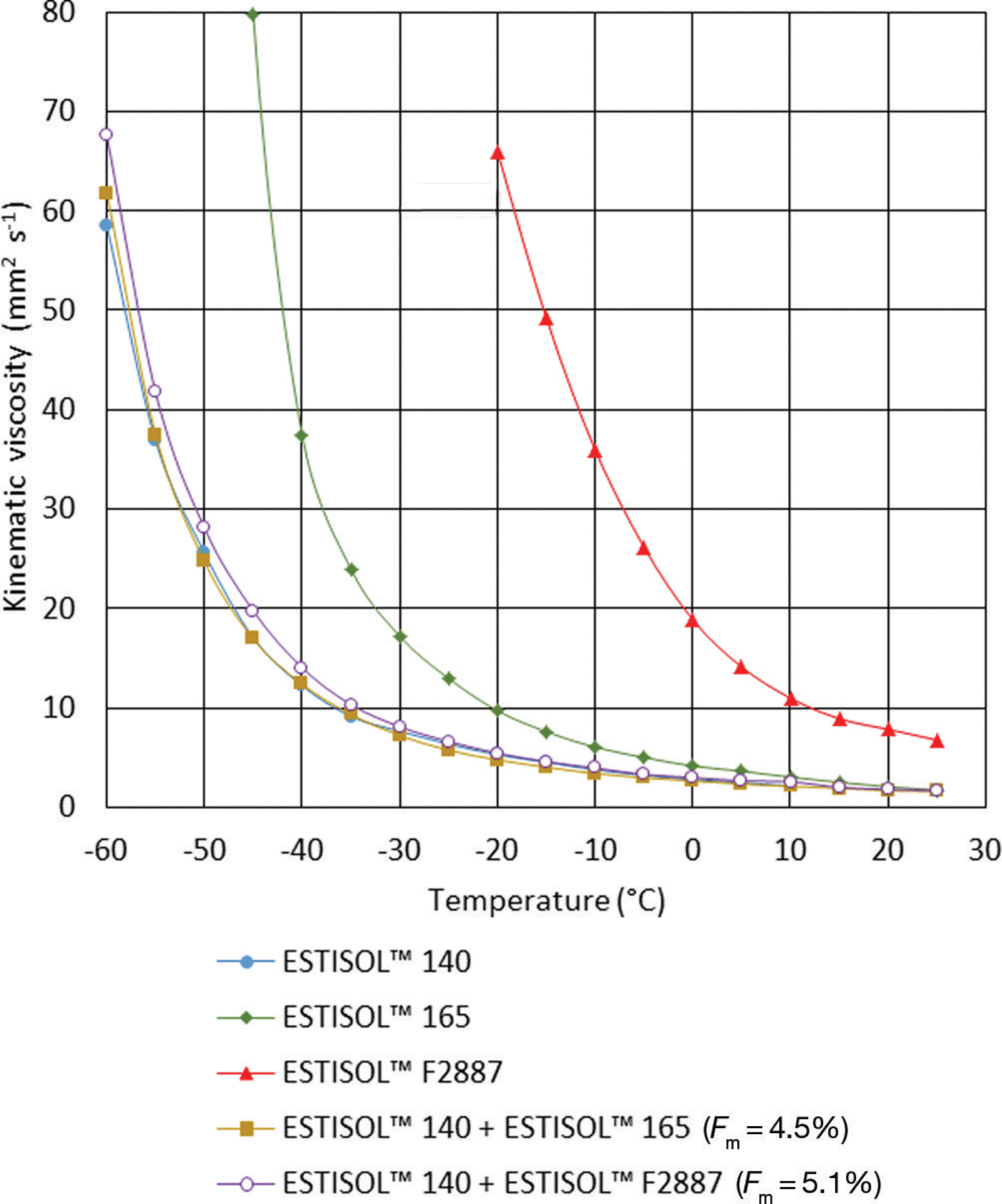
Fig. 2. Viscosity of single- and two-component ESTISOLTM esters vs temperature.
Table 5. Experimental viscosity–temperature equations of the single- and two-component ESTISOLTM esters under atmospheric pressure.

It should also be stated that the viscosity of ESTISOLTM 140 obtained in our experiments is higher than the experimental data from the Centre for Ice and Climate, University of Copenhagen (S. Sheldon and others, unpublished information), especially at low temperatures. They reported that the kinematic viscosity of this synthetic ester is 4.3 mm2 s–1 at -45°C, which is nearly three times lower than our results. This variation might be explained by the different experimental set-up used to obtain the data.
In May-June 2012, Danish specialists drilled a test borehole at an interval of 103-131 m at the North Greenland Eemian Ice Drilling (NEEM) camp, northwest Greenland, using a Hans Tausen electromechanical drill with single ESTISOLTM 140 fluid (S. Sheldon and others, unpublished information). A drill descent speed of 1.1 ms–1 was easily achieved in liquid at a temperature of ~–298C within the nominal clearance of 5.8 µm between the borehole walls and the outer barrel of the drill. Ice core of excellent quality was recovered, and no problems were encountered with cleaning and processing of the core as excess liquid evaporated quickly from the core surface. In general, the field tests proved the applicability of ESTISOLTM 140 for deep drilling in ice sheets.
Dimethylsiloxane oils
Low-molecular dimethylsiloxane oils (DSOs) can also be considered as a good alternative for borehole fluids, and are discussed at length elsewhere (Reference TalalayTalalay, 2007). Low-molecular DSOs are clear, colorless, tasteless, odorless and chemically neutral liquids. They are hydrophobic and essentially inert substances that are stable in contact with water, air, oxygen, metals, wood, paper and plastics. From the wide range of DSOs, two grades of silicones (KF96-1,5cs and KF96-2,0cs) most closely fit the requirements for drilling fluids.
With respect to accessing Vostok Subglacial Lake, DSO was planned to be delivered by special tanker to the bottom of the drilled deep borehole 5G-1 (Reference VerkulichVerkulich and others, 2002). It was anticipated that, being heavier than the kerosene-based drilling fluid and lighter than water, this hydrophobic liquid would create a 100 m thick ecologically friendly ‘buffer layer’ at the bottom of the hole. Unfortunately, due to logistical issues, the plan was not fulfilled and in February 2012 subglacial water was reached with jet fuel TS-1 mixed with HCFC 141b (Reference VasilievVasiliev and others, 2012). As low-molecular DSOs have never been used in ice-drilling projects, it is not possible to determine their suitability for deep drilling of ice. This can only be determined after field experiments have been conducted in a test borehole.
Environmental Issues of Low-Temperature Drilling Fluids
Occupational safety and health
Occupational safety and health considerations should address the physical, chemical and biological factors external to all types of workers employed as drillers, scientists, technicians and support personnel, and all the related factors affecting their health. Occupational safety and health encompasses the assessment and control of environmental factors that can potentially affect health. It is targeted towards preventing disease and creating healthy supportive environments.
During drilling, a large volume of drilling fluid is circulated in an open or semi-enclosed system with agitation, providing significant potential for chemical exposure and subsequent health effects. Severe health problems can occur if an employee inhales or absorbs harmful chemicals from drilling fluids through his or her mouth or nose, or via skin contact. The most dangerous chemical hazard from drilling fluids is usually from inhalation and skin contact.
The workplace air contaminant levels of vapour or gas in indoor air listed in Table 1 should be avoided in working rooms at a temperature of 25°C and pressure of 101 325 Pa, or protective equipment should be provided and used. The permissible exposure is specified as a time-weighted average (TWA) for a normal 8hour working day/40 hour working week, to which nearly all workers may be exposed repeatedly without adverse effect.
Kerosenes are suspected carcinogens. Human systematic effects occur by ingestion and intravenous routes, causing hallucinations and distorted perceptions, coughing, nausea or vomiting, and fever. Excessive exposure to jet fuels, which are used as base components of kerosene-based drilling fluids, may cause irritation to the nose, throat, lungs and respiratory tract. Central nervous system effects may include headache, dizziness, loss of balance and coordination, unconsciousness, coma, respiratory failure, and even death (www.hovensa.com/pdf/jeta.pdf). Jet fuels are severe skin irritants: contacts with skin may lead to irritation, infection and dermatitis.
Aromatic hydrocarbons with low boiling point (e.g. benzene, toluene and xylene) are more dangerous. The content of aromatics in EXXSOLTM D-series and IsoparTM K solvents is <0.1–0.6%, compared with the concentration of aromatics in jet fuels of 20–25%, which is why these solvents are considered less harmful than other kerosenes. Nevertheless, vapor concentrations above the exposure limit can cause eye and lung irritation and may result in headaches, dizziness or drowsiness (www.exxonmobilchemical.com/Chem- English/Files/Resources/exxsol-d40-fluid-product-safety-summary-en.pdf). Prolonged or repeated skin contact in an occupational setting may result in irritation.
Fluorocarbons were introduced in the 1940s as inert harmless agents, but later toxicological data showed that they are not inert and are slightly or moderately toxic if ingested or inhaled. Most fluorocarbons have similar patterns of toxicity. At high concentrations, fluorocarbons cause adrenaline to sensitize the heart so that arrhythmia may develop. Long-term exposure to high concentrations of this class of chemicals may cause adverse effects on the liver, nervous system and reproductive development.
Ethanol is a confirmed carcinogenic, tumorigenic and teratogenic material and is mildly toxic by inhalation. Ethanol has shown experimental reproductive effects, and human mutation data have been reported. Exposure to concentrations over the recommended threshold limit may cause headache and irritation of the eyes, nose and throat, and, if continued for an hour, drowsiness and lassitude and loss of appetite and concentration. Ethanol is also an eye and skin irritant.
n-Butyl acetate is a confirmed teratogenic material and is mildly toxic by inhalation and ingestion. n-Butyl acetate odors are detectable at 10ppm. Human systematic effects occur by inhalation, causing conjunctiva irritation and unspecified nasal and respiratory system effects. n-Butyl acetate is a skin and severe eye irritant and a mild allergen, and high vapor concentrations will cause narcosis. Recommendations of n-butyl acetate exposure limits adopted in different countries vary from 40 to 200 ppm (Reference Talalay and GundestrupTalalay and Gundestrup, 2002). Personal communications from PICO, Japanese and Chinese drillers indicated that after a workday in a drilling shelter, workers felt symptoms of the central nervous system (dizziness, headaches). It is impossible to use n-butyl acetate without sufficient ventilation and some means of removing the n-butyl acetate vapors from inhaled air.
ESTISOLTM esters are not considered hazardous according to the calculation procedure of the ‘General classification guideline for preparations of the EU [European Union]’ (safety data sheets for ESTISOLTM 140, ESTISOLTM 165 and ESTISOLTM F2887 provided by Esti Chem A/S, Gadstrup, Denmark). They are not classified (i.e. hazardous) substances for transport by road or air cargo, and do not present an explosion hazard. ESTISOLTM esters do not contain any relevant quantities of materials that have to be monitored in the workplace. The permissible exposure to ESTISOLTM esters in indoor air of the work area is not specified. So far, during the use of ESTISOLTM 140 in limited field experiments in Greenland a fruit-like odor was noted. However, even at subzero temperatures in the range –10°C to –25°C inside the drilling trench, appropriate ventilation would be desirable.
COASOLTM is also not classified as dangerous according to European Communities criteria. Based on test results, contact with the eye may cause mild irritation if the material is not washed from the eye, and prolonged exposure may cause skin dryness (http://www.dow.com/productsafety/pdfs/233-00694.pdf). COASOLTM tends to cause swelling and degradation of certain elastomeric polymers including natural rubbers, and field tests revealed that rubber-soled shoes were quickly destroyed following spillage of a mixture of ESTISOLTM 240 and COASOLTM on the floor of a drilling shelter.
DSOs are inert liquids that are acceptable for use as ingredients in cosmetics, for preventing human skin from chafing and as defoamers for food and beverages (Reference TalalayTalalay, 2007). There are no adopted workplace air DSO contaminant levels and, as a result, there are no recommendations for the control of workplace contaminant concentrations. Shin-Etsu Chemical Co., a DSOs manufacturer, notes only one potential hazard to human health: in contact with the eye, low-molecular DSOs cause temporary irritation but not permanent harm.
Ozone depletion and global warming
Reference Molina and RowlandMolina and Rowland (1974) drew attention to the potential biological hazard resulting from depletion of the ozone layer due to release of fluorocarbons into the atmosphere. No matter how paradoxical it appears, discovery of the annual depletion of ozone above the Antarctic was first announced in May 1985 (Reference Farman, Gardiner and ShanklinFarman and others, 1985), 5 years after the first use of CFC-11 at Vostok station (Reference Ueda and TalalayUeda and Talalay, 2007).
According to the Montreal Protocol, chlorofluorocarbons listed in Group I (CFC-11, CFC-113) were totally phased-out by 1996. The Montreal Protocol placed HCFC-141b, recently used as a densifier of kerosene-based fluids, on its Class II substance list. Originally, Class II compounds were designated for restrictions starting in 2015 and outright prohibition by 2030. Some countries have accelerated that process: in North America the US Environmental Protection Agency (EPA) forced a phase-out of HCFC-141b in 2003, and in Europe HCFCs have been banned since 2004. At this time HCFC-141b is produced mostly in developed countries, where consumption and production of HCFC should halt in 2013. These countries will cap and reduce the production and consumption of HCFC-141b gradually, starting in 2015, but it is still currently available on the market in China, South Africa and a few other countries.
Fourth-generation foam-expansion agents are characterized not only by zero ozone depletion potential (ODP) but also by lower global warming potential (GWP) compared with HFCs. Recent estimates indicate that DuPontTM FEA-1100 has a very short atmospheric lifetime of ~ 1 6 days.
Chemical pollution
Drilling fluids can contaminate large quantities of air, the surface and near-surface snow-firn layer, ice cuttings and, potentially, subglacial water resources. Chemical pollution is closely connected with drilling fluid processing (Fig. 3) as, at every step of processing, fluid is lost due to evaporation and spills (Reference YuYu and others, 2013). In contrast to regions with temperate climates, the biodegradation of drilling fluids is not likely to occur in regions with polar climates, as chemical dissolution of materials by bacteria or other biological means is almost impossible. The effects of drilling fluids are particularly important if fluid remains in the borehole; because of the movement of the ice, fluid in the hole will eventually reach the sea after a period of many thousands of years.

Fig. 3. Schematic diagram of drilling fluid circulation system (red arrows show drilling fluid losses): A: preparation section; B: pumping section; C: recycling section; 1: containers for components of drilling fluids; 2 : container for recycled drilling fluid; 3 : coarse-mesh filter; 4: hydro extractor; 5: mixing tank; 6: fine filter; 7: casing.
Air chemical pollution results from the release of drilling fluid compounds into the atmosphere. Contamination of air comes from evaporation of drilling fluid while it is prepared and spilled. At the particular location of the drilling site, the emissions would be temporary, and the total air quality impacts from drilling activities are likely to be small. The main problem of air contamination concerns toxicological and hazard control of the workplace. The high volatility of drilling fluids raises human health questions. For liquids with a high rate of vaporization it is necessary to carefully control air contamination and ensure that the threshold limits in air and the minimal inflammable concentrations are not exceeded. From this perspective, use of fluids with a low rate of vaporization is preferable.
Contamination of surface and near-surface snow–firn layers can result from two independent processes: (1) spillage of drilling fluid during processing and (2) drilling fluid losses in the borehole. It is well known that the upper part of the ice sheets comprises a permeable snow–firn layer, the thickness of which depends on the accumulation rate and temperature. In different regions of inland Antarctica, the depth of the firn–ice transition varies in the range 64–115 m (Reference PatersonPaterson, 1994). In order to reduce loss of the drilling fluid, the permeable snow–firn zone is isolated with casing in drilling operations. To prevent leaks at the bottom of the casing, a special shoe is installed (Reference Johnsen, Gundestrup, Hansen, Schwander and RufliJohnsen and others, 1994); however, the safety of the standard construction of the casing joint and casing shoe is not always sufficient to prevent fluid leakage. Problems with leakage of drilling fluid in different parts of the casing almost always become apparent while drilling (Reference Talalay and GundestrupTalalay and Gundestrup, 2000; Reference Vasiliev, Lipenkov, Dmitriev, Podolyak and ZubkovVasiliev and others, 2007).
Ice-cutting contamination is particularly important when considering the burial of cuttings. According to Article 2 of the Protocol on Environmental Protection to the Antarctic Treaty, waste like contaminated ice cuttings ‘shall be cleaned out or removed from the Antarctic Treaty area’. In spite of the fact that ice cuttings were polluted by drilling fluid, in recent drilling projects they have been buried near the drilling site and this will have local physical or chemical effects on the ice-sheet status. The greatest concern associated with such impacts is the possible adverse effects on future science efforts in the same location.
Contamination ofsubglacial water resources occurs when a body of water is adversely affected by the addition of drilling fluid, which is not a naturally occurring substance in aquatic ecosystems and causes degradation of ecosystem functions. The contamination effect depends on the solubility in water of components of the drilling fluid. Oil hydrocarbons, fluorocarbons, n-butyl acetate, ESTISOLTM esters, silicon oil and some drilling fluids are hydrophobic liquids in which water wets a liquid boundary surface to some extent, and after physical mixing promoted by flow turbulence the water and the liquid form an emulsion. Emulsions may separate into oil and water again under calm conditions. Chemicals that are lighter than water will accumulate on the base of the ice sheet, while heavy compounds will settle on or in the subglacial till or sediments and harm the ecosystem there. Aromatic compounds are more soluble in water than saturated hydrocarbons; therefore, creatures may be poisoned without direct contact with the oil by the polluted water. Water-soluble pollutants (i.e. ethanol) are miscible (or dissolve) in water due to their chemical composition, and the harmful effect depends on the pollutant concentration.
There are currently no references on environmental monitoring of several of the deep-drilling projects that have been completed at various sites on the Antarctic and Greenland ice sheets, and follow-up studies have not been conducted on the impacts of earlier drilling operations. According to measurements made by one of the authors (P. Talalay) during deep drilling in NGRIP-2, the volume of drilling fluid pumped into the hole from the surface was 21.3 L m–1, of which the volume of drilling fluid required to fill the drilled-in interval of the hole was 13.2 Lm–1. Recycling of the drilling fluid at the surface reduced drilling fluid consumption to 16.5 Lm–1. Wastage of completely lost drilling fluid therefore amounted to 3.3 Lm–1, and the volume rate of recycled drilling fluid was 4.8 Lm–1.
Summing up, it is apparent that during drilling of the NGRIP-2 borehole ~10m3 drilling fluid was lost at this site. Unfortunately, it is not possible to estimate how much of the drillingfluid wastage contaminated the air or snow-firn layer. The contamination of ice cuttings can be determined. The average weight of cuttings after the hydro extractor was 5.74-5.79 kg m–1 (Reference Talalay and GundestrupTalalay and Gundestrup, 2000) and the weight of the dry cuttings should be equal to 5.18 kg m–1. Subtracting the latter value from the former gives us the amount (~0.6kgm-1) of drilling fluid still contained in the cuttings, and it was not possible to extract more fluid from the cuttings by centrifugal force. Consequently, during drilling of the NGRIP-2 borehole ~15 tons (16.3 m3) of cuttings were buried at the drill site, which contain ~1.8 m3 drilling fluid.
Biological pollution
The interaction of drilling activity on the Antarctic ice sheet with surface or air biota is unlikely, but the impact of drilling fluid on subglacial water biota can occur at almost any inland drilling site. Biological pollution can be considered from two points of view: (1) the poison effect from components of the drilling fluid; and (2) ingress into the subglacial water of modern microflora from the surface.
Kerosenes are very harmful to microbiota. Even at very small concentrations in the aquatic environment (~1 mgm–3) the aromatic hydrocarbon component can be poisonous to microorganisms. On the other hand, a microbiological study of kerosene-based drilling fluid sampled at different depths from 110 to 3600 m in the 5G borehole at Vostok station showed that the drilling fluid itself contained bacteria of different origins, such as the ‘ubiquitous and omnivores’ genus Sphingomonas, a well-known degrader of aromatic hydrocarbons, and human and animal pathogens (Reference Bulat, Vasilieva, Petit, Lukin and AlekhinaBulat and others, 2003). The number of microbial cells in the lower, relatively warm (-6ºC to -10ºC) part of the borehole was found to be ~100cells mL–1 (Reference Alekhina, Vasiliev and LipenkovAlekhina and others, 2012).
Molecular microbiology studies of the frozen subglacial water samples recovered from the NGRIP-2 borehole filled with kerosene-based drilling fluid showed the presence of microbial DNA and a few phylotypes of bacteria, but the origin of these microorganisms is questionable as the samples were heavily contaminated by drilling fluid (Reference Bulat, Alekhina, Petit, Steffensen and Dahl-JensenBulat and others, 2005). When the subglacial water first entered the borehole, it contacted and mixed with the toxic drilling fluid, and it is almost impossible to get uncontaminated samples out of a borehole that is stabilized with kerosene-based drilling fluid (Reference WilhelmsWilhelms, 2007).
Unfortunately, most of the other low-temperature drilling fluids have the same toxic properties. For example, ethanol is a well-known antiseptic used as a bactericide and fungicide. It kills microorganisms by denaturing their proteins and dissolving their lipids, and is effective against most bacteria and fungi and many viruses (Reference McDonnell and RussellMcDonnell and Russell, 1999). However, ethanol is ineffective against bacterial spores, which can remain in ethanol-water solution in great numbers.
The effects and associated toxicological mechanisms of esters on microorganisms have been confirmed by experiments (e.g. Reference Zhou, Cai and XingZhou and others, 2011), but the specific effects on microbiota of n-butyl acetate and ESTISOLTM esters should be investigated in case these liquids are to be considered for future use in accessing subglacial lakes. Silicone oils are not toxic to microorganisms; however, tested microorganisms showed an apparent decrease in colony-forming units in silicone oils (Reference Özdamar, Araz, Ozturk, Akin, Karacorlu and ErcikanOzdamar and others, 1999; Reference Mackiewicz, Kozioé-Montewka, Kosior-Jarecka, Szczepanik, Wójtowicz and ZagórskiMackiewicz and others, 2004).
In order to avoid the potential for biological contamination of the subglacial environment and subsequent impact on microbial function, before dumping drilling fluid into the hole the fluid should be carefully treated on the surface and microbial contamination control methods should be introduced. Sterilization of drilling fluid is necessary for the complete destruction or removal of all microorganisms (including spore-forming and non-spore-forming bacteria, viruses, fungi and protozoa) that could contaminate subglacial water. In principle, drilling fluid should be cleaner than subglacial water. The microbial populations of Vostok Subglacial Lake are currently unknown, but can be predicted from research on accretion ice at Vostok station, where the concentration of microorganisms was found to be 24-100 cells mL–1 (Reference Alekhina, Vasiliev and LipenkovAlekhina and others, 2012).
Standard sterilization methods include heating, filtration and UV treatment. Some aspects of sterilization methodology have already been developed for hot-water ice-drilling technology and the proposed exploration of Ellsworth Subglacial Lake (Reference Mowlem, Siegert, Kennicutt and BindschadlerMowlem and others, 2011) and Subglacial Lake Whillans (Reference Fricker, Siegert, Kennicutt and BindschadlerFricker and others, 2011). It should be noted that many of these methods are not suitable for sterilization of organic drilling fluids; for example, the filtration of kerosene-based drilling fluids through fine microbiological membranes is not possible as components from the drilling fluid form films, which completely block the membrane pores (Reference Bulat, Vasilieva, Petit, Lukin and AlekhinaBulat and others, 2003). Heat treatment of water and chemicals also differs in procedure, temperature and duration (Reference Reichert and YoungReichert and Young, 1993). Therefore, development of new protocols will be required for sterilization of low-temperature drilling fluids.
Conclusions
The search for a new environmentally friendly drilling fluid for coring in central Antarctica remains one of the most pressing problems for future drilling projects, including those projects intending to access subglacial lakes. Hot water is considered by the scientific community as the cleanest drilling fluid medium, but it cannot solve the environmental problems completely because modern microorganisms contained in snow-ice can ingress into the subglacial water. Furthermore, the thermal pollution of hot-water circulation could immensely influence the viability of subglacial microorganisms.
Not all recent low-temperature drilling fluids (kerosene-based fluids, ethanol and n-butyl acetate) qualify as intelligent choices from the safety, environmental and technological standpoints. It is likely that the next stage of evolution of ice-drilling fluid technology will develop with the introduction of synthetic ESTISOLTM esters, which are non-hazardous substances. ESTISOLTM 140 mixtures with ESTISOLTM 165 and ESTISOLTM F2887 have an acceptable density and viscosity at low temperatures, and field tests have proved ESTISOLTM 140 applicability for deep drilling in ice sheets. Low-molecular DSOs can also be considered a good alternative for borehole fluids, but the final conclusion about the applicability of DSOs in deep ice drilling can be made only after field experiments in a test borehole.
If ESTISOLTM esters or DSOs are planned for use to access subglacial lakes then the specific harm for all types of microorganism should first be investigated. In addition, a new protocol should be developed to regulate sterilization of the drilling fluid on the surface before dumping into the borehole.
Acknowledgements
This paper describes the research conducted under the general project ‘Research on environmentally friendly drilling fluid impact on Polar regions environment’ (No. 41276189) organized by the National Science Foundation of China, and the non-profit project ‘Technology of wall stability retention in deep boreholes drilled in ice sheets’ (No. 201311041) granted by the Ministry of Land and Resources of China. We thank Alex Pyne and Robyn McFarlane of the Antarctic Research Centre, Victoria University of Wellington, New Zealand, for very helpful comments and for editing this paper, and anonymous reviewers for helpful advice.