Introduction
Ongoing losses in global biodiversity as a result of the overuse or underuse (e.g. land abandonment) of land by human populations necessitate increased efforts to conserve species across broad spatial scales. Variability in the patterns of biodiversity across spatial scales has been a focus of conservation ecology in recent decades (Summerville et al., Reference Summerville, Boulware, Veech and Crist2003; Coetzee et al., Reference Coetzee, Gaston and Chown2014). These patterns are regulated by multiple environmental variables over fine and broad scales (Groves et al., Reference Groves, Jensen, Valutis, Redford, Shaffer and Scott2002; Luoto et al., Reference Luoto, Virkkala and Heikkinen2007). The identification of important spatial scales that support all elements of species diversity is essential for the effective allocation of limited conservation resources (Jost et al., Reference Jost, DeVries, Walla, Greeney, Chao and Ricotta2010). Although broad-scale conservation studies that incorporate geographical variability have become more common (Crist et al., Reference Crist, Veech, Gering and Summerville2003; Gering et al., Reference Gering, Crist and Veech2003; Müller & Goßner, Reference Müller and Goßner2010), there have been few investigations of species diversity patterns at national or continental scales (Luoto et al., Reference Luoto, Virkkala and Heikkinen2007). Broad-scale conservation is especially important for the preservation of species diversity in remnant and fragmented ecosystems, including fragmented forests, remnant grasslands and montane moorlands (Wagner et al., Reference Wagner, Wildi and Ewald2000; Martin et al., Reference Martin, Moloney and Wilsey2005; Sasaki et al., Reference Sasaki, Katabuchi, Kamiyama, Shimazaki, Nakashizuka and Hikosaka2012).
Species-rich, semi-natural grasslands were maintained for centuries by human management activities (e.g. burning, mowing, grazing); their abandonment during shifts to modern agricultural practices has increased fragmentation and reduced the landscape areas under grass (Kahmen et al., Reference Kahmen, Poschlod and Schreiber2002). Although preservation and restoration efforts aimed at conserving biodiversity have made some progress in remnant semi-natural grasslands (MacDougall & Turkington, Reference MacDougall and Turkington2007), most of these efforts have been applied locally (i.e. single grasslands), without considering regional or national scales. Local-scale preservation and restoration efforts often focus only on the responses of particular threatened species (e.g. local Red Listed species) (Vellak et al., Reference Vellak, Tuvi, Reier, Kalamees, Roosaluste, Zobel and Pärtel2009), without taking account of net species diversity losses (Lennon et al., Reference Lennon, Beale, Reid, Kent and Pakeman2011; Chiarucci et al., Reference Chiarucci, Bacaro, Filibeck, Landi, Maccherini and Scoppola2012). Although plant species diversity in semi-natural grasslands has been well studied at diverse spatial scales (Hansson & Fogelfors, Reference Hansson and Fogelfors2000; Myklestad & Sætersdal, Reference Myklestad and Sætersdal2004; Auestad et al., Reference Auestad, Rydgren and Økland2008), identification of important scales for effective conservation of all species is notably lacking.
The areal extent of semi-natural grasslands in Japan has declined rapidly since the 1960s because of abandonment, land development and the proliferation of coniferous plantations (Takahashi, Reference Takahashi2004; Ogura, Reference Ogura2006; Nagata & Ushimaru, Reference Nagata and Ushimaru2016); these grasslands now comprise < 1% of the nation's total land area. Montane semi-natural grasslands exist in isolation across Japan, and most have been preserved and designated as national parks or quasi-national parks.
Our aim was to identify important spatial scales for conserving entire species complements, thereby facilitating the development of an effective nationwide strategy for the preservation of remnant semi-natural grasslands. Additive partitioning of species diversity is a useful approach for quantifying the spatial patterns of biodiversity sampled in hierarchically scaled studies (Lande, Reference Lande1996; Veech et al., Reference Veech, Summerville, Crist and Gering2002; Crist et al., Reference Crist, Veech, Gering and Summerville2003). This approach facilitates additive partitioning of the total diversity in an area into scale-specific diversity components (Wagner et al., Reference Wagner, Wildi and Ewald2000; Veech et al., Reference Veech, Summerville, Crist and Gering2002; Gering et al., Reference Gering, Crist and Veech2003) and identifies the spatial scale most relevant to the conservation of the entire species complement. We applied procedures for additive partitioning of diversity to vegetation datasets assembled for representative montane semi-natural grasslands across Japan. Species diversity patterns across spatial scales may vary with the geographical range of individual plant taxa (i.e. whether they are widespread or narrowly distributed; Crist et al., Reference Crist, Veech, Gering and Summerville2003; Gering et al., Reference Gering, Crist and Veech2003; Müller & Goßner, Reference Müller and Goßner2010). Thus, we aimed to identify the most important elements of overall species diversity, with a particular focus on grassland species and Red Listed species. We discuss appropriate spatial scales for the allocation of preservation and restoration efforts that best conserve species complements nationwide.
Study sites
The study was conducted in 11 montane semi-natural grasslands across Japan (Table 1; Fig. 1), excluding the islands of Okinawa and Hokkaido, spanning most of the representative, historically managed montane grasslands in the country. The grasslands (Aso, Shiozuka, Akiyoshi-dai, Mt Ungetsu, Mt Sanbe, Hiruzen, Soni, Asagiri, Sengokuhara, Minakami and Kawatabi) are located in a range of climatic zones, from warm-temperate to cool-temperate. All of the locations were managed through regular spring burning and/or seasonal mowing procedures. In some of the grasslands (e.g. Mt Ungetsu, Sengokuhara, Minakami), management activities were suspended temporarily after World War II and/or the earthquake disaster in 2011 but had been resumed 2–10 years prior to the study. The grass Miscanthus sinensis Andersson predominates in continually managed grasslands in Japan (Koyanagi et al., Reference Koyanagi, Kusumoto, Hiradate, Morita, Yokogawa, Takahashi and Sato2013); Pleioblastus argenteostriatus (Regel) Nakai and Sasa veitchii (Carrière) Rehder were also dominant in some of the grasslands studied.
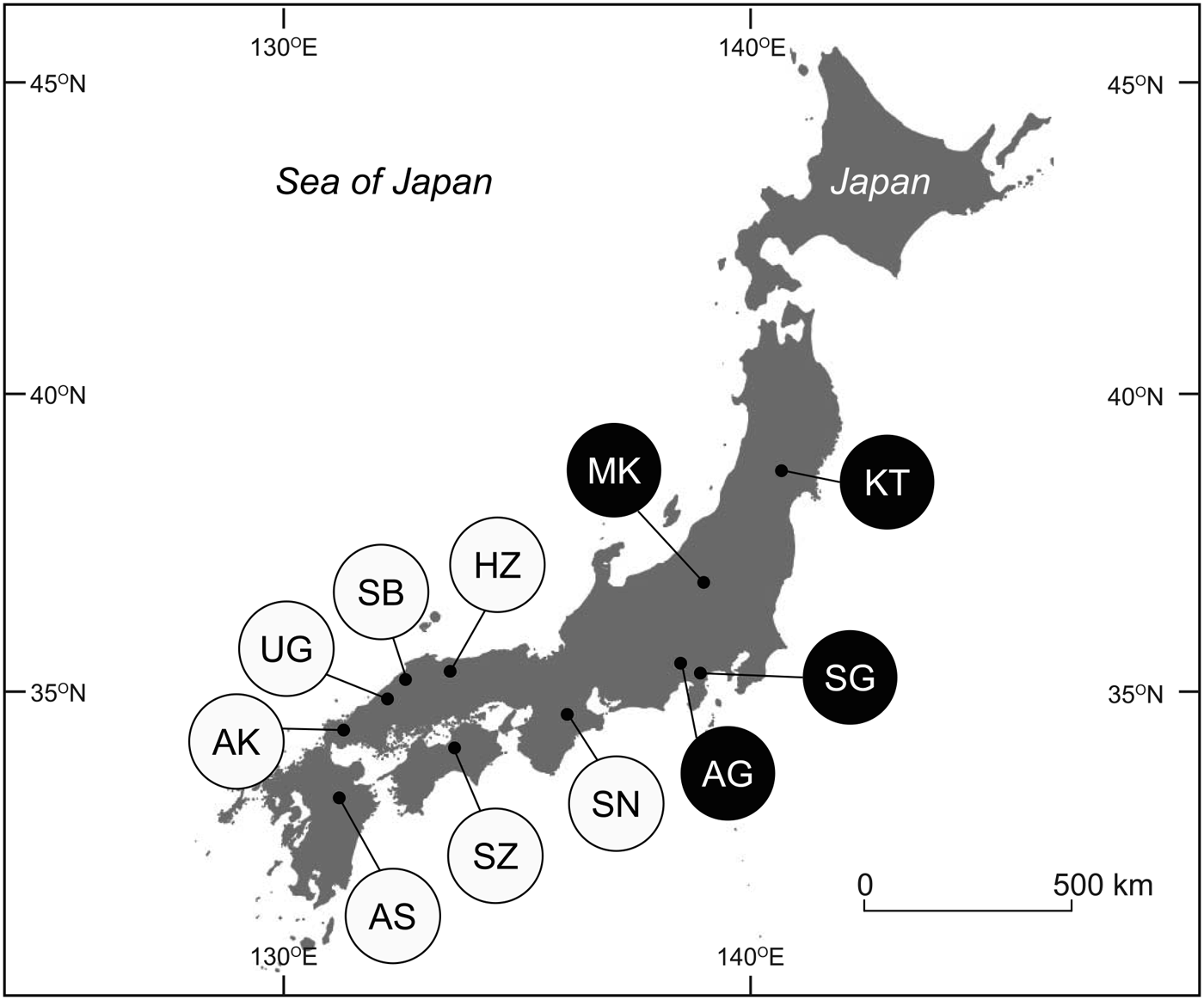
Fig. 1 Locations of the 11 semi-natural grassland study areas in Japan. White circles, western Japan; black circles, eastern Japan. See Table 1 for site codes.
Table 1 Details of the 11 semi-natural grassland study sites in Japan, with codes (Fig. 1), number of quadrats, mean number of species per m2, area, elevation, and whether or not burning and mowing are carried out.

* The mown area was not identified.
Methods
Survey design
During June–September in 2010, 2011 and 2015 we conducted vegetation surveys in each grassland. We set 24–74 quadrats (1 × 1 m2) at random, according to the area of each grassland, deployed across a range of topographies. Characteristic species in M. sinensis-dominated communities were categorized as grassland taxa (Koyanagi & Furukawa, Reference Koyanagi and Furukawa2013). Red Listed species of vascular plants were identified using the national Red List (Ministry of the Environment, Government of Japan, 2012) and the Red Lists for the 45 prefectures, to include the entire range of listed species (excluding those on Okinawa and Hokkaido). We extracted species categorized as Extinct, Extinct in the Wild, Critically Endangered, Endangered, and Vulnerable from these Red Lists. Red Listed tree and shrub species (21 species) were not included in the study.
The vegetation data were compiled in a hierarchical nested matrix (Table 1). The three hierarchical levels corresponded to the following spatial scales: regional (western vs eastern Japan), grassland (0.1–220 km2), and quadrat (491 quadrats, each 1 m2). The plants were grouped into three categories: all species, grassland species and Red Listed species. At the regional scale the variation in the geological structure of the Japanese islands, which are split by the Fossa Magna rupture zone (Maekawa, Reference Maekawa1949), was evident. The western and eastern regions included seven and four grasslands, respectively.
Statistical analysis
To determine the adequacy of our sampling we computed species rarefaction curves for separate grasslands and for all grasslands pooled (491 quadrats in total), with a Monte Carlo permutation of samples (Kobayashi, Reference Kobayashi1973; Chiarucci et al., Reference Chiarucci, Bacaro, Rocchini and Fattorini2008), using the specaccum routine in the vegan package of R v. 3.0.3 (Oksanen et al., Reference Oksanen, Blanchet, Kindt, Legendre, Minchin and O'Hara2016). In each case a mean species accumulation curve was constructed from data collected by sub-sampling the entire community 1,000 times without replacement. Although studies have demonstrated that spatial autocorrelation needs to be incorporated in the calculation of sample-based rarefaction curves (Chiarucci et al., Reference Chiarucci, Bacaro, Rocchini, Ricotta, Palmer and Scheiner2009; Bacaro et al., Reference Bacaro, Altobelli, Cameletti, Ciccarelli, Martellos and Palmer2016), our data are not suitable for this spatially explicit method because of a lack of coordinate data for some quadrats. Therefore, we used this result only for confirmation of data sampling. We then estimated total species richness non-parametrically with first-order jackknife estimates using the equation:

where n is the total number of samples, S is the number of species observed in n quadrats, and r1 is the number of species present in only one quadrat (Palmer, Reference Palmer1990).
The contributions of the various spatial components to overall species diversity were quantified by additive partitioning of species richness (Lande, Reference Lande1996; Crist et al., Reference Crist, Veech, Gering and Summerville2003). In this approach the entire diversity of a sampling unit (γ) can be separated into additive components: α (within sampling units) and β (between units) diversity (Allan, Reference Allan1975; Lande, Reference Lande1996). This additive procedure can be extended across multiple scales in a hierarchical spatial sampling design (Crist et al., Reference Crist, Veech, Gering and Summerville2003). We partitioned total plant diversity across grasslands nationwide into the diversities contributed by each of the spatial scales:

We used species richness as the diversity index (Jost et al., Reference Jost, DeVries, Walla, Greeney, Chao and Ricotta2010) for the three plant groups (all species, grassland species and Red Listed species).
To test the significance of level-specific α and β estimates of diversity partitioning, we tested a null model hypothesis using the adipart function in the vegan package (Oksanen et al., Reference Oksanen, Blanchet, Kindt, Legendre, Minchin and O'Hara2016) through a sample-based randomization procedure. The null model hypothesis prediction that species diversity was uniform at all spatial scales was examined by additive partitioning of the total diversity (γ diversity). We used a complete randomization procedure (Crist et al., Reference Crist, Veech, Gering and Summerville2003), repeated 1,000 times to obtain null distribution of each α and β estimate for plant species diversity at each spatial scale. Each of the original level-specific estimates was then compared with the appropriate null distribution and used to test the null hypothesis that the observed α and β diversities were obtained from a random distribution of individuals among samples at all hierarchical levels. Statistical significance was assessed from the proportions of null values that were greater or smaller than the actual estimates. We are aware that the additive partitioning method across spatial scales has an issue with the lack of independence of β and α diversity components (Jost, Reference Jost2007; Schmera & Podani, Reference Schmera and Podani2013). Nevertheless, the additive method is useful for estimating the contribution of various levels of the sampling hierarchy to overall diversity, and is widely used (Bacaro et al., Reference Bacaro, Maccherini, Chiarucci, Jentsch, Rocchini and Torri2015). For future analyses, more promising approaches consider pairwise dissimilarities and are based on partitioning the dissimilarity matrix of sampling units (Schmera & Podani, Reference Schmera and Podani2013).
Results
We recorded 390 plant species in total (excluding 48 unidentified species) from the 491 quadrats; the mean number of species was 5.6–20.6 (Table 1). These included 120 grassland species and 171 Red Listed species (66 species in common; Supplementary Table S1). The number of species accumulated increased with the number of quadrats sampled, and the curves tended to become saturated towards the maximum number of sampling units (Fig. 2). The first-order jackknife estimate of total richness for all grasslands was 469 (Fig. 2b). The observed richness indices in individual grasslands and across all grasslands were > 75 and > 82% of the estimated total, respectively (Fig. 2a,b).

Fig. 2 Species accumulation curves (a) for each grassland site, and (b) fitted to all sites. Asterisks indicate the first-order jackknife estimates of total richness. See Table 1 for site codes.
The additive partitioning of species diversity produced consistently higher α 1, β 2 and β 3 diversity values than expected by chance for all three plant groups (all species, grassland species and Red Listed species) but the β 1 diversity value was lower than would be expected by chance (Fig. 3). Thus, a non-random ecological process determined species distributions across spatial scales. Differences among grasslands (β 2) accounted for the largest proportion of total species richness for the three plant groups across spatial scales. We also found differences between grassland species and Red Listed species in terms of the proportions of total diversity accounted for by different spatial scales. The second-highest proportions for all species and Red Listed species were accounted for by differences between regions (β 3), but the highest proportion for grassland species was accounted for by differences among quadrats (β 1).

Fig. 3 Additive partitioning of diversity. Proportion of total species diversity accounted for by α and β components at three spatial scales (regions, grasslands and quadrats), for (a) all species, (b) grassland species, and (c) Red Listed species. +, the observed value is statistically higher than the expected; −, the observed value is statistically lower than the expected; ***, P < 0.001.
Discussion
We found that differences among grasslands (β 2) accounted for the largest proportion of total species diversity in remnant semi-natural grasslands across Japan. Thus, non-random ecological processes operating at the among-grassland scale were important determinants of plant species composition and richness. Previous studies have shown that broad spatial scales (e.g. the regional scale) are the most relevant for the conservation of insect assemblages in forest ecosystems (Gering et al., Reference Gering, Crist and Veech2003; Müller & Goßner, Reference Müller and Goßner2010) and plants in protected areas or fragmented farmlands (Flohre et al., Reference Flohre, Fischer, Aavik, Bengtsson, Berendse and Bommarco2011; Chiarucci et al., Reference Chiarucci, Bacaro, Filibeck, Landi, Maccherini and Scoppola2012). However, intermediate-scale diversity (e.g. between-plot diversity) is the most relevant for the total species diversity of woody plants in temperate forest ecosystems (Chandy et al., Reference Chandy, Gibson and Robertson2006). We found that between-region effects were not the largest contributors to overall diversity; among-grassland effects were larger, suggesting that each grassland has a unique grouping of species. In our dataset 186 species (including 24 grassland species and 81 Red Listed species) were recorded from only a single grassland (Supplementary Table S1). Therefore, conserving beta diversity in each grassland is essential for preserving nationwide plant species diversity in semi-natural grasslands.
The contribution of regional-scale effects to overall species diversity is determined by differences in climate, biogeographical factors, historical processes, and dispersal limitation between regions (Gering et al., Reference Gering, Crist and Veech2003; Müller & Goßner, Reference Müller and Goßner2010). The relatively high contribution of β diversity among grasslands (β 2) in our study was probably a result of multiple factors, such as soil type, historical land-use, geographical variation, grassland extent, and seed-dispersal limitation among grasslands. Soil type determines the plant composition and richness of semi-natural grassland communities (Gibson et al., Reference Gibson, Seastedt and Briggs1993; Kahmen et al., Reference Kahmen, Perner, Audorff, Weisser and Buchmann2005; Auestad et al., Reference Auestad, Rydgren and Økland2008). The soils of some of the semi-natural grasslands we studied included limestone (e.g. Akiyoshi-dai) and volcanic ash (e.g. Aso and Sengokuhara). Historical land-use also determines the plant species richness of grassland communities (Hansson & Fogelfors, Reference Hansson and Fogelfors2000). In totally abandoned grasslands (e.g. Sengokuhara and Minakami) the species richness of grassland taxa and Red Listed species has decreased (Grman et al., Reference Grman, Bassett and Brudvig2013), probably as a result of increased cover of woody plants (Pykälä et al., Reference Pykälä, Luoto, Heikkinen and Kontula2005). Geographical factors, particularly elevation and aspect, regulate temperature and solar radiation, and can thus determine the distribution of grassland species of northern origin (Marini et al., Reference Marini, Scotton, Klimek, Isselstein and Pecile2007). The extent of each grassland, in combination with geographical factors (e.g. elevation, slope angle and aspect), is another principal determinant of species turnover (Bennie et al., Reference Bennie, Hill, Baxter and Huntley2006; Marini et al., Reference Marini, Scotton, Klimek, Isselstein and Pecile2007; Yamato et al., Reference Yamato, Ema and Takeda2013); however, areal estimation is difficult in mosaic and isolated Japanese grasslands (Table 1). In the largest semi-natural grassland in Japan (Aso) we found several endemic species and 119 Red Listed species, including four categorized as Vulnerable at the national level (e.g. Trigonotis radicans Steven and Silene sieboldii (Van Houtte) H. Ohashi & H. Nakai; Supplementary Table S1). Because the distribution of remnant semi-natural grasslands in Japan is limited, identifying the principal determinants of species differences among them is difficult. More detailed studies on the combined effects of soil type, human land-use, grassland extent and geographical factors on plant species diversity should improve understanding of the uniqueness of individual grasslands.
We found that the second-ranked spatial scale contributing to overall species diversity differed between grassland species and Red Listed species. The second-ranked spatial scale for grassland species was among quadrats (β 1), whereas for all species and Red Listed species, between-regions diversity (β 3) ranked second among the contributors. Previous studies (Crist et al., Reference Crist, Veech, Gering and Summerville2003; Gering et al., Reference Gering, Crist and Veech2003, Gabriel et al., Reference Gabriel, Roschewitz, Tscharntke and Thies2006, Sasaki et al., Reference Sasaki, Katabuchi, Kamiyama, Shimazaki, Nakashizuka and Hikosaka2012) have shown similar patterns of species partitioning (i.e. widespread distributions of common species, and regionally specific distributions of rare species). Most grassland species are typical of semi-natural grasslands and occur widely in this ecosystem across Japan. Miscanthus sinensis, Lysimachia clethroides Duby, Potentilla freyniana Bornm. and Ixeridium dentatum (Thunb. ex Thunb.) Tzvelev occurred in all 11 grasslands that we studied. However, species richness within quadrats was highly variable, ranging from 4 (Kawatabi) to 14 (Mt Sanbe) for grassland species (Fig. 2). Grassland management activities (e.g. burning and mowing) increase habitat heterogeneity within grasslands, thereby enhancing local species diversity (Uchida & Ushimaru, Reference Uchida and Ushimaru2015). Topographical variability within grasslands may also relate to the impact of burning or mowing and help maintain high diversities of grassland species at small spatial scales. Unlike the grassland species, most Red Listed taxa are by definition narrowly distributed; threatened species include local endemics and relic species. Adenophora triphylla (Thunb.) A.DC. var. triphylla was recorded only in the grasslands of western Japan (Supplementary Table S1). The proportions of various spatial scales contributing to diversity in the ecosystem studied were similar for Red Listed species and the overall community complement, implying that Red Listed species drive the patterns of the entire community (Gering et al., Reference Gering, Crist and Veech2003; Müller & Goßner, Reference Müller and Goßner2010). Species conservation efforts that make use of area protection protocols, such as those used for national parks, are often considered to be inadequate for the preservation of narrowly distributed species (Gaston & Fuller, Reference Gaston and Fuller2009; Watson et al., Reference Watson, Evans, Carwardine, Fuller, Joseph and Segan2011). Our findings indicate that grassland species and Red Listed species are probably best protected by different conservation strategies. The conservation of grassland species requires ongoing routine management within each grassland, but the conservation of Red Listed species calls for the maintenance of the unique attributes of each grassland within a regional perspective.
Implications for biodiversity conservation
Our findings emphasize the need for a nationwide perspective on the conservation of plant diversity in remnant semi-natural grasslands. A nationwide approach is beginning to develop in Japan, mostly in relation to bird communities within forest and grassland ecosystems (Yamaura et al., Reference Yamaura, Ikeno, Sano, Okabe and Ozaki2009; Katayama et al., Reference Katayama, Amano, Naoe, Yamakita, Komatsu and Takagawa2014). Although the remnant montane semi-natural grasslands of Japan (most of which are located within national parks or quasi-national parks) are protected from land development, regular maintenance (i.e. burning and mowing) has ended or declined as the human population has decreased and managers have aged. The high risk of accidents caused by burning, and the high cost of continuing management activities have also accelerated the abandonment of semi-natural grasslands (Valkó et al., Reference Valkó, Török, Deák and Tóthmérész2014). We found that preserving the beta diversity of individual grasslands is indispensable for nationwide conservation of plant species diversity. Thus, we suggest several potentially effective nationwide approaches. Firstly, a compensatory practice of offsetting species diversity losses in particular grasslands by gains in other locations (Kiesecker et al., Reference Kiesecker, Copeland, Pocewicz, Nibbelink, McKenney and Dahlke2009, Reference Kiesecker, Copeland, Pocewicz and McKenney2010), implemented in some countries, is not a viable strategy (Apostolopoulou & Adams, Reference Apostolopoulou and Adams2017). The species diversities of individual grasslands cannot be replaced by diversities of other grasslands because each grassland is unique. Secondly, restoration efforts should be allocated broadly across remnant semi-natural grasslands to boost the numbers of grassland and Red Listed species. Thirdly, strengthening regional cooperation is essential for effective and efficient conservation (Pouzols et al., Reference Pouzols, Toivonen, Di Minin, Kukkala, Kullberg and Kuusterä2014), especially for Red Listed species. These nationwide perspectives on diversity conservation strategies will be useful for conservation efforts in other remnant and isolated ecosystems. In conclusion, we have highlighted the importance of strengthening local preservation and restoration activities within each grassland, and nationwide strategies for conserving Red Listed species in remnant semi-natural grassland communities.
Acknowledgements
We are grateful to Yu Yoshihara (Mie University) for providing field data, and to Yoshiko Kobayashi (Hokkaido University), Takeshi Osawa (National Institute for Agro-Environmental Sciences), Taiki Masui (Gifu University) and the members of the grassland research group in western Japan for their help on our field survey. Dr Martin Fisher and two anonymous reviewers provided valuable comments. This work was supported financially by Environment Research and Technology Development Funds (D-1001, 1-1401). MA was funded by the Japan Society for the Promotion of Science (no. 25740048).
Author contributions
AK, TFK and MA conceived and designed the surveys and analysed the data. AK, TFK, YK and SH conducted the surveys. All authors contributed to the writing of the article.
Biographical sketches
Asuka Koyama is a plant ecologist, whose research interests include biodiversity conservation and ecological management. Tomoyo Koyanagi is a landscape ecologist interested in landscape changes of semi-natural grasslands, and the efficient use of traditional knowledge and plant resources. Munemitsu Akasaka’s work focuses on planning for biodiversity conservation and biological invasions. Yoshinobu Kusumoto is an expert in vegetation science, and researches the environmental assessment of agricultural and grassland ecosystems. Syuntaro Hiradate is a specialist in soil analysis. Masayuki Takada’s work focuses on environmental assessment and environmental policy, particularly in relation to wetland and grassland ecosystems. Kimiko Okabe is an ecologist and manages several research projects on biodiversity evaluation and biological invasions.