In Part IFootnote * of this series of articles P. D. Baird has described the method he used to measure the accumulation of superimposed ice ; the difficulties of obtaining an average value for an area will have been realized. In this paper several further items of equipment and techniques used on the Barnes ice cap are described. The experiences taught many lessons and an account of them may be of assistance to other glaciologists when working beyond the reach of normal resources.
Thermo-electric Boring in Cold Ice
A thousand feet of aluminium alloy tube, in. (73 mm.) external diameter and
in. (63 mm.) internal diameter supplied by the Canadian Aluminum Co., a 2 kilowatt 115 volt petrol-electric generator supplied by the Fairbanks Morse Co., and 1000 ft. (305 m.) of heavy conductor cable were taken to the ice cap. Our intention was to sink the tube into the ice to measure its vertical velocity and temperature distribution. The unexpectedly low temperature and the imperviousness of the ice unfortunately prevented any great depth being reached by the tube.
The thermo-electric boring method was developed by J. F. Gerrard at Cambridge, England, for use in ice at its melting point and has been used by Perutz,Reference Perutz 4 SharpReference Sharp 7 and NizeryReference Nizery 2 in temperate glacier studies. The Baffin equipment was similar to earlier models in its original design, except that the tip heater was placed inside the lower closed end of the aluminium tube, so that the heater could be removed if necessary. Aluminium tube had the advantage of lightness and permitted the use of a simple magnetic inclinometer. The tube was supplied in ten foot (3 m.) lengths which were screwed together with a bituminous jointing compound to form perfectly flush and watertight joints.
The heat was originally concentrated at the tip of the tube, two types of heater being used. On an energy basis, one seemed as successful as the other. One heater consisted of a commercial sealed tubular heater (115 volts, 1,200 watt) about 6 in. (15 cm.) long and 1 in. (2.5 cm.) diameter. This was fitted into the centre of a thick copper bit to form, in effect, a large soldering iron that was slid inside the aluminium tube (see Fig. 1a , p. 118). The other unit (sec Fig. 1b ) was designed and made by the Physics Department of McGill University, with the assistance of Mr. J. D. C. Waller of the Engineering Department. It consisted of a copper core wound externally with a single spiral of resistance wire about 25 ft. (7.6 m.) long, (2000 watts at 115 volts) insulated with ceramic fish spines. The extended tip of the copper core slid into a hole in the aluminium tip to give good thermal contact. Initial trials of both heaters were made by Mr. Waller at McGill University.
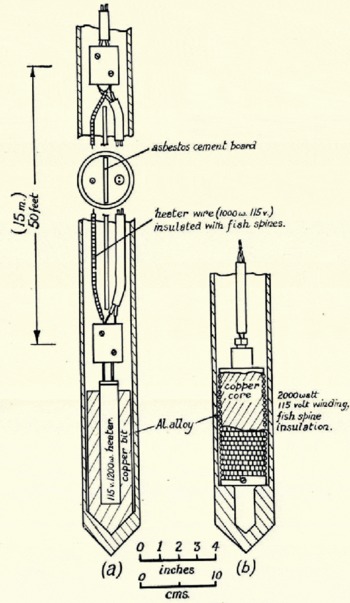
Fig. 1. Thermo-electric boring tube
Both units were tested early in June at Camp A.1 for the purpose of setting a few thermistors permanently in the ice. The tube equipped with the 1200 watt soldering iron heater froze in a depth of 10 ft. (3 m.) and was melted out by slowly moving the heater up and down the tube. By continually rotating and moving the tube up and down, a much greater depth was reached with the 2000 watt heater. Further progress became impossible at 30 ft. (9.1 m.) depth and it was rapidly lifted out of the ice. No melt-water was lost from either hole and in the 30 ft. deep hole it froze within a few minutes of the tube being extracted. This unfortunately prevented a thermistor being lowered further than 13 ft. (4 m.).
The heater arrangement was then modified, so as to obtain a maximum penetration of the ice with the comparatively small amount of energy and the limited resources available. The soldering iron heater was placed at the tip of the tube and a spare 50 ft. (15.3 m.) length of resistance wire (1000 watts at 115 volts), threaded with fish spines, was connected in parallel. This 50 ft. (15.3 m.) long heater was stretched out up the tube alongside the rubber-insulated cable, but separated from it by an equal length of asbestos cement board (see Fig. 1a ).
This new arrangement was quite difficult to erect and was sunk into the ice to a depth of 70 ft. (21.3 m.) at Camp A.2X early in July. It was finally left there with thermistors frozen inside. A penetration of 35 ft. (10.7 m.) was made in 12 hours at a generator potential of 120 volts when signs of the tube freezing in were noticed. The generator speed was increased to give a potential of 140 volts and a further penetration of 35 ft. (10.7 m.) was obtained in the next 13 hours. Despite a further rise in potential to 160 volts no further penetration was made, presumably because the tube had frozen to the ice above the top of the long heater. There was no loss of water from the hole and the tube was a close fit in the hole, except for a few feet near the surface where solar radiation caused local melting. The actual distribution of power used in the final stages of penetration amounted to about 1800 watts at the tip plus 30 watts per foot of tube for 50 ft. (15.3 m.). Thus the power necessary to penetrate the ice at a depth of 1000 ft. (300 m.) would need to be about 30 kilowatts, or rather less if allowance is made for the increase in temperature of the ice with depth.
Finally, in trying to extract the heaters it was unfortunate that some of the many lengths of asbestos cement board jammed together inside the tube and caused the cable to part at the junction with the top of the 50 ft. heater. This prevented thermistors from being lowered into the tube to a depth greater than 30 ft.
It is evident that the task of sinking a long tube into low temperature impervious ice is quite a formidable one. If a series, instead of a parallel circuit, is used, some form of long insulated resistance cable is required which is preferably in good thermal contact with the tube. It would be convenient if the full length of heater cable could be initially coiled inside the lower part of the tube so that it became uncoiled and extended as further lengths of tube are added. Another possibility is to use the tube itself as a resistance element; this would need an insulated cable inside. that withstands heat, and a variable output generator.
Mechanical Drilling in Cold Rock and Cold Ice
The “X-ray” petrol-driven diamond drilling unit, manufactured by Boyles Bros. Drilling Co., Ltd., and loaned to the expedition by the Canadian Defence Research Board, was used both for cold rock drilling and, with a special coring tool, for drilling in cold ice. Practical hints on the use of the drill were provided by Mr. J. P. Croal before departure.
(a) Cold Rock
Gneiss, cropping out as a small shelf on a hillside by Generator Lake (see Part II, Fig. 1, Journal of Glaciology, Vol.2, No. 11, p. 13) was drilled. It had a steeply dipping structure and its temperature decreased from about +2° C. at the surface to about −9° C. at z5 ft. (4.6 m.) deep. The standard diamond coring barrel drilling a hole 1.19 in. (3 cm.) diameter was used, but a separate gasoline-driven gear pump fed the drill with water from the lake below (temperature never more than +5° C.). Normal drilling progress was maintained to a depth of 15 ft. (4.5 m.), without the core barrel freezing in, so long as the work was uninterrupted. The water froze in the hole overnight, but either salt was added or the ice was jetted away with water in the morning. Between 18 ft. and 25 ft. (5.5 and 7.6 m.) boring became more difficult ; sometimes when a core was lost it froze in and, in effect, the same ground had to be bored again.
It had been intended at this stage, when the water started to freeze, to change to kerosene as the drilling liquid, the kerosene being re-circulated to avoid waste of a liquid precious in these circumstances. Unfortunately this procedure was not feasible, for despite the massive nature of the rock, several large fissures between 4 and 8 ft. (1.2 and 2.4 m.) below surface lost water rapidly and caused springs some distance downhill. The short length of lining tube available was not long enough to seal the leak and during an attempt at further progress with water the coring barrel froze in and was lost. It is thought, however, that drilling with kerosene would have been possible.
A group of “Stantel” thermistors was finally lowered in the hole and sealed in with ice and waste paper.
Fig. 2 (p. 114) shows the drill in operation.

Fig. 2. “X-my” drill at Generator Lake (see text, p. II7)
(Photograph by M. H. W. Ritchie)
(b) Cold Ice
A short length of casing with a diamond-set shoe proved useless for drilling in cold ice. Practically no penetration was obtained even with a high axial load. Cold ice may be drilled with a saw-toothed coring tool, either dry or with a non-freezing liquid to flush the ice cuttings clear of the tool. The only difficulties with dry ice drilling are:
-
the removal of the ice cuttings away from the cutting edge so as to avoid jamming, and
-
the risk that surface melt water may descend and freeze the drill solid.
Diversion of the surface water is simple. Liquid drilling in remote areas is undesirable because of the transport of the liquid. Dry drilling was the only method attempted on the ice cap, and the work was entirely experimental since such cold dense ice near the surface was not anticipated.
The same “X-ray” drilling unit (weight 90 kg.) was used. It was equipped on the ice cap with its own broad skis fashioned from box-wood and bolted to the four legs. Thus two men could tow it quite readily anywhere on the cap, through slush rivers and across lake ice submerged in water. Little axial thrust was required when drilling, other than the weight of the unit and that of the drill rods (1.6 lb./ft., 2.4 kg./m.) even when running with the throttle full open and in top gear. Thus the problem of finding kentledge on the ice cap did not arise. It would have been better, however, if the machine could have been equipped with a slow speed reverse gear to give a much slower rate of extraction of the drill rods and so avoid stalling the engine when the coring tool tended to jam.
The coring tool worked surprisingly well in cold ice, seeing that it was intended for use in firn and melting ice. It tended to jam when due care was not taken and the cores were disturbed. Experience indicated some modifications and these are referred to below, since they may be of use in further developments. The tool was 1.5 in. (3.8 cm.) in diameter and similar to the one shown in Fig. 3 (p. 118), except that there was only one vertical slot in the wall instead of the four spiral slots; it was based on the experiences of Seligman.Reference Seligman 5 A much larger diameter tool could have been used in conjunction with the “X-ray” unit, if required. Cuts 15 to 18 in. (38 to 45 cm.) long could be made in ice at −12° C. with our tool, without any risk of jamming. The ice cuttings from the set saw-teeth found their way upwards into the slot and on to the top of the ice core. The core was easily released from the solid drawn steel tube by warming it for a moment in the engine exhaust gases. Occasionally there was difficulty in raising the coring tool, even though it was quite free to rotate. This is believed to be due to the whirling of the drill rods causing the sharp shoulder at the top of the coring tool to cut an overhanging ledge in the ice wall. This difficulty could be avoided by reducing the coring tool diameter almost to that of the drill rods (1.09 in., 2.8 cm.), but this reduces the clearance between the drill rods and the ice, and increases the risk of freezing up. Another possibility is to have another set of saw-teeth on the upper edge of the core barrel. In a limited trial the latter method seemed satisfactory. The spiral slots shown in Fig. 3 are suggested to improve the upward travel of ice cuttings from the saw-teeth and to prevent jamming of the core.

Fig. 3. Saw-toothed coring tool
In cold ice there was no risk of losing the core, but in melting ice it may be necessary to use a separate core picker as used in rock drilling. This consists of a plain tube with two short leaf springs inside at the bottom that grip the lost core when it slides in.
If a drilling liquid such as kerosene is used all slots must be omitted and the tube must extend well above the drill rod to the tube connection so as to collect the ice chips, like a calyx drill.
It is considered that the “X-ray” drill could be used conveniently for drilling to depths of about 150 ft. (46 m.) in cold ice. Down to this depth at least this type of drilling is likely to be more convenient than thermoelectric boring. Both methods need more development before their full advantages can be realized.
Measurement of Temperatures in Ice and Rock
In view of the writer’s previous experiences with thermistors and the simplicity and lightness of their recording equipment, they seemed the obvious choice for remote temperature measurements in the circumstances.
The thermistors were supplied by Standard Telephones and Cables Ltd., and are known as “Stantel” type KB2231/80 ; their resistance is about zoo ohms at +20° C. and 2000 ohms at −15° C. They are mounted on a metal plate and their overall size is about by
by
in. (32 by 22 by 6 mm.). Each one needs calibrating. In addition, the thermistors had to be,
-
mechanically and electrically attached to lengths of cable,
-
protected from damage and
-
insulated at the bare leads.
Fig. 4 (above) illustrates the metal capsules which were made for these purposes. Each thermistor was sealed in a short length of soft brass tube ; this was closed at both ends by nipping it in a bench vice and soldering the joints. The flattened tube capsule was filled with molten soft grease and the lead wires were soldered to a short length of plastic-covered twin-cored cable. The plastic cover was gripped by one closed end of the capsule to anchor it to the cable. These capsules were only used where slowly changing temperatures were to be recorded, as occurs in the ice. For rapid response to quickly changing temperatures, such as air temperatures, the capsule was omitted. Instead the cable was anchored to the metal mounting of the thermistor with a small strip of brass and the lead wires and connections were insulated in a shroud of Bostik 692 sealing compound applied in two coats. All cable connections made on the ice cap were soldered and insulated with the same compound and finally wrapped in adhesive plastic tape. With these precautions all the thermistors proved entirely satisfactory, including some that recorded in strong brine.

Fig. 4. Thermistor recording circuit and thermistor capsules
The Wheatstone bridge recorder, constructed by the author at the Building Research Station, was based on the design of Penman and LongReference Penman and Long 3 ; but was modified for lightness and according to equipment immediately available. The circuit is given in Fig. 4 and the recorder is illustrated in Fig. 5 (p. 114). It weighs 4.6 lb. (2.1 kg.). The fixed resistances are of cracked carbon type with a low temperate coefficient. The two variable arms of the bridge consist of two outer 300 ohm fixed resistances and a linear wire-wound potentiometer (205 ohm), which can be moved as a unit by double-pole double-throw switches (1, 2, 3 and 4) into four series positions between and on either side of three 200 ohm fixed resistances. This arrangement provides a variable arm ratio of 3:11 to 11 : 3, and gives accuracy combined with lightness. Two U.2 dry cells in series (3 volt) provide the bridge current and one pair of these cells lasted the whole summer. The galvanometer has a sensitivity of about 1 mm. per microamp. For safety, a 20,000 ohm resistance is kept permanently in series with the galvanometer; this resistance can be shorted by a spring-loaded push button B when balancing.

Fig. 5. Thermistor Recorder (see text, p. 120)
The recorder was calibrated against resistance and each thermistor was directly calibrated for temperature on the recorder. A table was drawn up of recorder readings for each 0.1° C. for an average thermistor. This table gave a rough value for the temperature of all thermistors, which was corrected by reference to a temperature correction curve plotted for each thermistor. At −25° C. 1° C. represented 20° rotation of the potentiometer and at +25° C. 1° C. represented 13° rotation. A set of four cartridge resistors, one balancing in each of the four switch positions, were used to check the calibration of the recorder in the field.
Snow Kit and Measurement of Ice Density
The snow kit was kindly supplied by the National Research Council of Canada (KleinReference Klein 1 ) and proved very practical in design. The sample cutters were neither suitable, nor intended, for coring ice and its density was measured from the weight of kerosene displaced by several lumps of ice from an improvised pycnometer jar. The density of the ice was always greater than the kerosene, and fresh kerosene, kept aside for the purpose, was used for each measurement.
The pycnometer was a peanut butter jar of about 270 cm.3 capacity with a convenient press-on self-sealing metal lid. A fine hole pierced in the lid enabled the same volume to be obtained for each test. The temperature of the kerosene was kept below o° C. and the lumps of ice added to the jar were weighed by difference, in case of melting in warm weather, on the snow kit balance.
Crystal Rubbings on Cold Ice
The paper and pencil rubbing technique (SeligmanReference Seligman 6 ), which is in common use on temperate glaciers, proved unsatisfactory on cold ice. It was tried several times with different grades of paper, hardness of pencil and surface preparation such as melting with a stove, irons, alcohol and the palm of the hand, but only air bubbles could be revealed readily on the paper. Yet the crystal boundaries could be seen easily by light reflected from a smooth surface and by the minute frost crystals that formed in damp weather on the exposed surfaces of the glacier crystals, but not so readily along their boundaries.
For rubbings in situ it was necessary to leave the excavation exposed to radiation in warm weather for several days. This caused preferential melting along the crystal boundaries sufficiently deep to be recorded by the pencil. It was generally quicker, however, to detach a block of ice and to leave it exposed to the sun or even the heat of the kitchen until the whole mass was melting.
Later in the summer when the ice was bare, reliable rubbings could be made on excellent natural surfaces, requiring no preparation, found beneath surface stones near the edge of the ice cap. The crevasses were generally too narrow to be accessible and the walls of melt water channels were difficult to reach. Moreover, in both these places, one could never be certain whether the ice was not recently refrozen melt water.
Rubbings on the buried glacier ice were made on excavated lumps left to melt in the sun. It is evident from these experiences that “rubbings” can only be made satisfactorily on melting ice.
Acknowledgements
The generous assistance and advice given by numerous firms, individuals and technical organizations, as well as the efforts of Mr. P. D. Baird in selecting and collecting much of the equipment are gratefully acknowledged. Mr. J. D. C. Waller assisted in the use of the equipment in the field.