Introduction
Obstructive sleep apnea (OSA) is a sleep-related breathing disorder characterized by repetitive complete (apnea) or partial (hypopnea) obstructions of the upper airway, resulting in oxygen desaturation and/or arousals from sleep (Reference Yeghiazarians, Jneid and Tietjens1;Reference Chowdhuri, Quan and Almeida2). Due to lifestyle, aging, and a rise in obesity, the prevalence of OSA has risen over the past few decades, with a reported prevalence of 33.9 percent in men and 17.4 percent in women aged 30–70 years in 2013 (Reference Peppard, Young and Barnet3). Established risk factors include male sex, older age, obesity, race/ethnicity, and family history (Reference Yeghiazarians, Jneid and Tietjens1). Untreated OSA has been associated with several cardiovascular (CV) sequelae, including hypertension, atrial fibrillation, heart failure, coronary artery disease, and CV mortality, in addition to impaired health-related quality of life and daytime sleepiness (Reference Yeghiazarians, Jneid and Tietjens1;Reference Kuhn, Schwarz, Bratton, Rossi and Kohler4).
OSA severity is assessed by the apnea-hypopnea index (AHI), which is the number of apnea and hypopnea episodes per hour during sleep. Mild OSA is defined as an AHI between 5 and <15 episodes per hour. A recent scientific statement from the American Heart Association recommends that all patients, including those with mild OSA, should be considered for treatment (Reference Yeghiazarians, Jneid and Tietjens1). Continuous positive airway pressure (CPAP) has long been considered the mainstay of OSA treatment (Reference Patil, Ayappa and Caples5). Oral appliances (OA) have provided an effective alternative for mild-to-moderate OSA and patients who cannot tolerate CPAP (Reference Patil, Ayappa and Caples5). Both therapies are effective but have been associated with significant long-term adherence challenges, especially in mild OSA (Reference Schwab, Badr and Epstein6–8). Further, nightly therapy compliance remains challenging, with patients on treatment often using their devices for only part of the night and thus not achieving the full theoretical treatment benefit (Reference Masa and Corral-Peñafiel9).
Neuromuscular electrical stimulation (NMES) is emerging as a noninvasive daytime therapy for mild OSA. Recently, daytime stimulation with a novel intraoral neuromuscular stimulation device (eXciteOSA®, Signifier Medical Technologies Ltd, London, UK) has been investigated for the treatment of mild OSA (Reference Kotecha, Wong, Zhang and Hassaan10;Reference Wessolleck, Bernd and Dockter11). NMES treatment with the eXcite OSA device involves noninvasive transoral stimulation for 20 min per day for a period of 6 weeks to increase the endurance of the tongue muscles to prevent the collapse of the tongue during sleep (Reference Kotecha, Wong, Zhang and Hassaan10;Reference Kotecha12;Reference Nokes, Baptista, de Apodaca, Carrasco-Llatas, Fernandez, Kotecha, Wong, Zhang, Hassaan and Malhotra13). Repeat treatment at specified follow-up periods is indicated to maintain the desired effect over time. In the studies conducted to date, NMES was found to be associated with improvement in objective and subjective measures of patient and bed partner sleep quality and patient daytime somnolence (Reference Kotecha, Wong, Zhang and Hassaan10;Reference Wessolleck, Bernd and Dockter11;Reference Nokes, Baptista, de Apodaca, Carrasco-Llatas, Fernandez, Kotecha, Wong, Zhang, Hassaan and Malhotra13). Due to the growing evidence that mild OSA is associated with clinical implications, healthcare stakeholders need to understand the clinical benefits and cost-effectiveness of available therapies. The objective of the current study was to conduct an exploratory analysis of the potential cost-effectiveness of NMES compared to CPAP, OA, and no treatment in the American healthcare system.
Methods
Overview
A Markov model previously published for evaluation of diagnostic and therapeutic strategies in OSA (Reference Pietzsch, Garner, Cipriano and Linehan14), and subsequently used to explore the cost-effectiveness of novel treatment modalities for moderate-to-severe OSA in the United States and Germany (Reference Pietzsch, Richter and Randerath15;Reference Pietzsch, Liu, Garner, Kezirian and Strollo16) was adapted for the current analysis, with the general model structure widely maintained as structure and events also apply to mild OSA. The analysis model tracked the progression of the cohort through relevant health states based on multivariable risk equations, with a lifetime horizon modeled based on the chronic nature of the condition and its treatment and the long-term risk implications of treatment. Event risks were adjusted to reflect those expected in a mild OSA population. The base case analysis assumed treatment was not associated with any CV benefit, whereas a second and exploratory scenario evaluated the implications of potential treatment-associated CV benefit. No institutional review board (IRB) or ethics committee approval was required for the study.
Model structure
The analysis model comprised five health states and tracked the monthly occurrence of stroke, myocardial infarction (MI), motor vehicle accidents (MVA), and death based on multivariable risk equations, and integrated poststroke and post-MI states to account for different mortality risks and costs. A schematic representation of the computational model is provided in the Supplementary Materials. Table 1 provides a summary of key model inputs. CV event risks in patients with mild OSA were assumed to be elevated compared to a general, non-OSA population. Specifically, a hazard ratio (HR) of 1.23 was applied for cardiac events that was calculated from regression analyses of data reported in a 10-year outcomes study, and an HR of 1.74 based on data from the Sleep Heart Health Study (Reference Marin, Carrizo, Vicente and Agusti21;Reference Redline, Yenokyan and Gottlieb30). As previously noted, the base case analysis did not assume any treatment-related reductions of CV risks, whereas a scenario analysis assumed some reduction of these risks, with each therapy’s effectiveness in reducing these excess risks estimated from the aforementioned regression analysis that linked AHI and CV risk (see supplementary materials and prior publication) (Reference Pietzsch, Liu, Garner, Kezirian and Strollo16). Under therapy compliance, the calculated reduction in excess risk was 59.2 percent for NMES (based on an AHI reduction from 10.2 to 6.8), 57.6 percent for OA (based on an estimated reduction in AHI from a common baseline of 10.2 to 7.0 events per hour, derived from effectiveness reported in the TOMADO trial (Reference Quinnell, Bennett and Jordan34), assuming nightly compliance with therapy), and 100 percent for CPAP (based on an estimated reduction in AHI from common baseline of 10.2 to 1.4 per hour, derived from effectiveness reported in the MERGE trial, assuming nightly compliance with therapy) (Reference Wimms, Kelly and Turnbull33). These effectiveness assumptions were further adjusted through multiplication with therapy-specific adherence. The proportion of the cohort assumed to be nonadherent returned to baseline risk levels identical to those incurred by untreated patients, as no subsequent treatment pathways were modeled after stopping one particular treatment.
Table 1. Input parameters
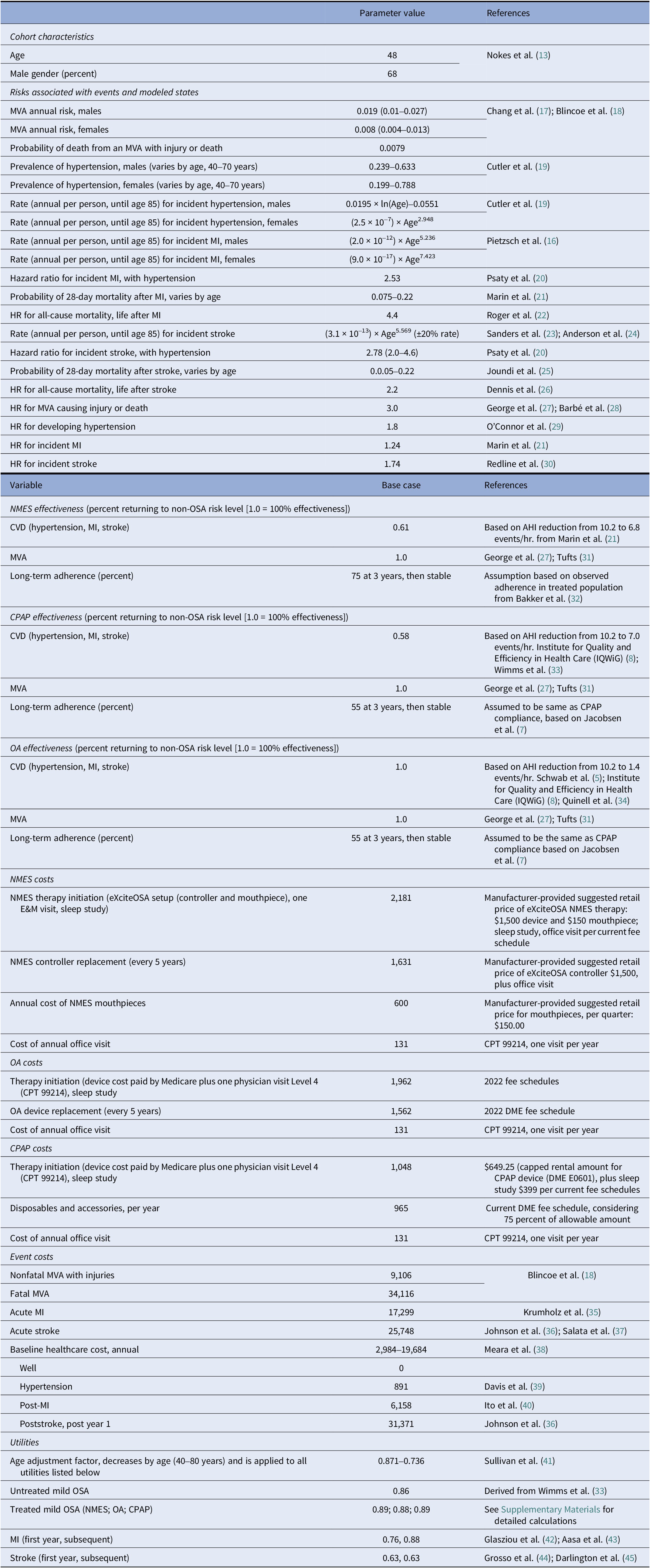
Clinical data
Cohort characteristics were derived from the mild OSA subcohort (N = 65) of a recent multicenter study of participants treated with NMES (Reference Nokes, Baptista, de Apodaca, Carrasco-Llatas, Fernandez, Kotecha, Wong, Zhang, Hassaan and Malhotra13). In this cohort (mean age 48 years, 68 percent male gender), the mean baseline AHI of 10.2 events per hour was reduced to 6.8 events per hour (Reference Nokes, Baptista, de Apodaca, Carrasco-Llatas, Fernandez, Kotecha, Wong, Zhang, Hassaan and Malhotra13). For the “no treatment” strategy, patients were assumed to remain at the baseline AHI of 10.2. To identify effectiveness assumptions for the CPAP and OA comparator strategies, the findings of a recent systematic search were used (8). Limiting the studies identified in that search to those with a mean baseline AHI of 15 events per hour or less yielded one study each for CPAP and OA––the MERGE trial and the TOMADO study (Reference Psaty, Furberg and Kuller33;Reference Cutler, Sorlie and Wolz34). MERGE was a multicenter, parallel, randomized controlled trial that enrolled patients from 11 UK sleep centers with an AHI of 5–15 events per hour which closely resembled the cohort characteristics of the NMES study. In MERGE, 115 patients were allocated to CPAP and N = 118 to standard care (Reference Wimms, Kelly and Turnbull33). At a mean follow-up of 3 months, the CPAP group reduced their AHI from baseline of 10.6 to 1.5 events per hour while on therapy. The TOMADO study was a crossover randomized controlled trial studying OA use in 83 patients with OSA (Reference Quinnell, Bennett and Jordan34). The mean pretreatment AHI of 13.8 events per hour was reduced to 9.5 events per hour. Cohort characteristics of the MERGE and TOMADO studies were closely comparable to the NMES cohort (see Supplementary Materials). The long-term therapy adherence for CPAP was assumed to be 55 percent, as reported at 3 years for the mild OSA subcohort in a retrospective study of N = 695 newly diagnosed OSA patients (Reference Jacobsen, Eriksen and Hansen7). Based on a study that demonstrated comparable adherence between OA and CPAP over time, OA was assumed to have the same long-term adherence as CPAP. For NMES, a recent study reported that the study population completed a therapy session on 83 percent of available days, over an average follow-up period of 6 weeks (13;32). Building on this information and the fact that NMES is used during the day, the analysis explored a base case scenario of 75 percent long-term adherence for NMES, and a low adherence (LA) scenario of 65 percent.
In the scenario analysis that explored the potential effects of cardiovascular benefit, CPAP was assumed to reduce OSA-associated cardiovascular risk completely in the base case. Additional computations were performed for assumed lower cardiovascular risk reduction benefits of only 80 and 60 percent that might be expected if part of the CPAP-adherent patients did not use their device for the full night, and a scenario that considered only 50 percent of the CV benefit calculated for the respective therapy.
Health-related quality of life
Estimates for health-state-specific utility values were based on data from the published literature. Values ranged from 0.63 (poststroke) to 1.0 (asymptomatic patient with no CV morbidity and a nonelevated AHI before age adjustment) (see Table 1). Utility estimates for untreated, mild OSA were derived from the MERGE study, which collected baseline EQ-5D values for patients with mild OSA (Reference Wimms, Kelly and Turnbull33). Study-reported improvements in the Epworth Sleepiness Scale (ESS) were used to calculate EQ-5D improvements based on a previously published algorithm (Reference McDaid, Griffin and Weatherly46). See Supplementary materials.
Resource use and costs
The NMES treatment cost, whilst not yet fully established in the American healthcare system, was based on assumptions about therapy cost (controller and mouthpiece) and, where applicable, existing reimbursement rates (e.g., home sleep test conducted prior to therapy initiation). Importantly, in the absence of allowable reimbursement amounts, NMES device costs were informed by manufacturer-suggested retail prices. OA and CPAP treatment costs––conversely––were based on current Medicare fee schedules as a proxy for true cost. For CPAP accessories/disposables, 75 percent of the maximum allowable monthly cost was used in the base case based on expert interviews. For NMES, OA, and CPAP strategies, a device lifetime of 5 years was considered, after which the devices would be replaced at the expense of Medicare. See Table 1 for detailed assumptions.
The model accounted for both acute event costs (MI, stroke, and MVA) and health-state-specific costs. All costs were derived from published literature and publicly available data (see Table 1). The consumer price index inflator was used to adjust historical costs to 2021 costs where applicable. Both costs and outcomes were discounted at 3.0 percent per annum, in line with recommendations for health-economic analysis (Reference Sanders, Neumann and Basu23).
Model outcomes and willingness-to-pay threshold
The primary analysis outcomes were strategy-specific total costs, quality-adjusted life year (QALY) gains, and the resulting incremental cost-effectiveness ratio (ICER), measured in dollars per QALY gained over the patient’s lifetime. In line with recommendations by the joint statement of ACC/AHA on cost-effectiveness, an intervention with an ICER of up to USD150,000 per QALY gained was considered “of value” (Reference Anderson, Heidenreich and Barnett47). Consequently, a willingness-to-pay threshold of USD150,000 per QALY gained was applied to evaluate cost-effectiveness.
Scenario, sensitivity, and threshold analyses
In addition to the base case analysis and the exploratory scenario that considered CV benefit, several other scenarios were calculated. These included calculations for a hypothetical cohort aged 65 years to more closely resemble a Medicare population. To evaluate the effect of variation in therapy adherence, CPAP/OA adherence and NMES adherence were varied between 100 and 45 percent, and 100 and 55 percent, respectively, in two-way sensitivity analyses. These were performed to determine which of the strategies were preferred for different combinations of therapy adherence assumptions, with preference determined based on a willingness-to-pay threshold of USD150,000 per QALY gained. Where this two-way analysis considered CV benefit, three assumptions of CPAP-associated CV risk reduction were explored to reflect partial compliance in CPAP-adherent patients. These scenarios included a 60, 80, and 100 percent effectiveness assumption resulting from only partial therapy use during the night. Further, in addition to the 75 percent Medicare allowable amount for CPAP accessories/disposables, 50 and 100 percent were explored as scenarios.
A range of one-way sensitivity analyses were conducted to evaluate the effect of parameter uncertainty on analysis results. A sensitivity analysis was calculated to determine cost assumptions where NMES might become cost-saving versus CPAP therapy, on the grounds that NMES costs assumed in the current analysis may be higher than negotiated Medicare rates.
Results
Compared to no treatment, NMES was the preferred treatment option, adding 0.30 QALYs (13.57 versus 13.26) and USD17,445 in costs (USD527,853 versus USD510,407) over the patients’ lifetime, resulting in an ICER of USD57,219 per QALY gained (Table 2). For the NMES low adherence scenario, the ICER was minimally higher at USD57,844 per QALY gained, based on 0.27 (13.53 versus 13.26) QALYs gained and incremental costs of USD15,498 (USD525,905 versus USD510,407), rendering NMES a cost-effective intervention. Relative to CPAP, NMES added 0.07 QALYs (low adherence scenario 0.03 QALYs) at an added cost of USD3,291 (USD1,324), leading to a cost-effective ICER of USD44,528 (USD36,160) per QALY (Figures 1 and 2). For the older treatment age of 65 years, NMES versus no treatment resulted in an ICER of USD71,312 (low adherence scenario USD72,198), NMES versus OA in an ICER of USD73,301 (USD79,990), and NMES versus CPAP in an ICER of USD66,335 (USD67,973) per QALY gained. See Table 2 for full results reporting of scenario analyses.
Table 2. Base case and scenario analysis results
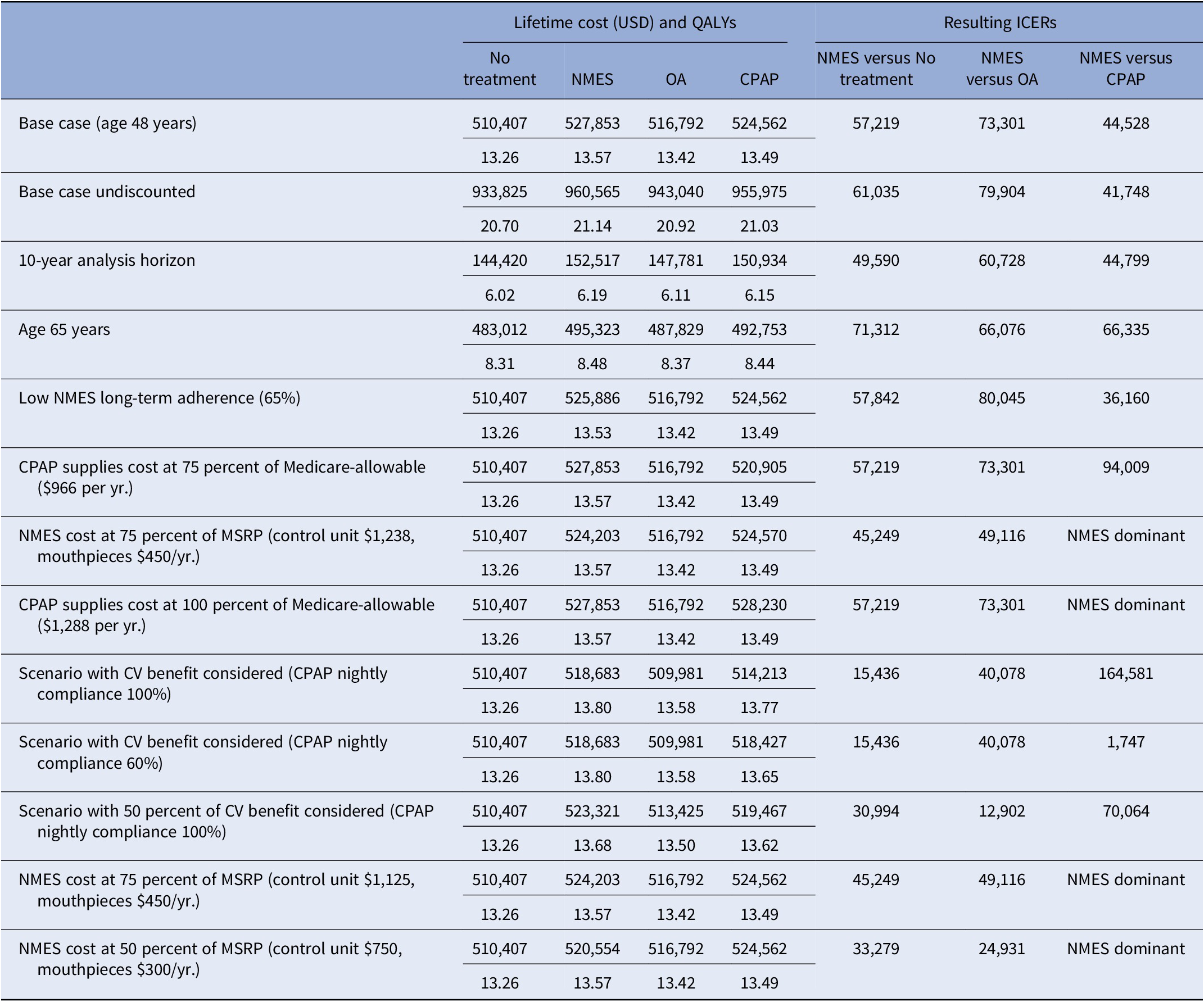
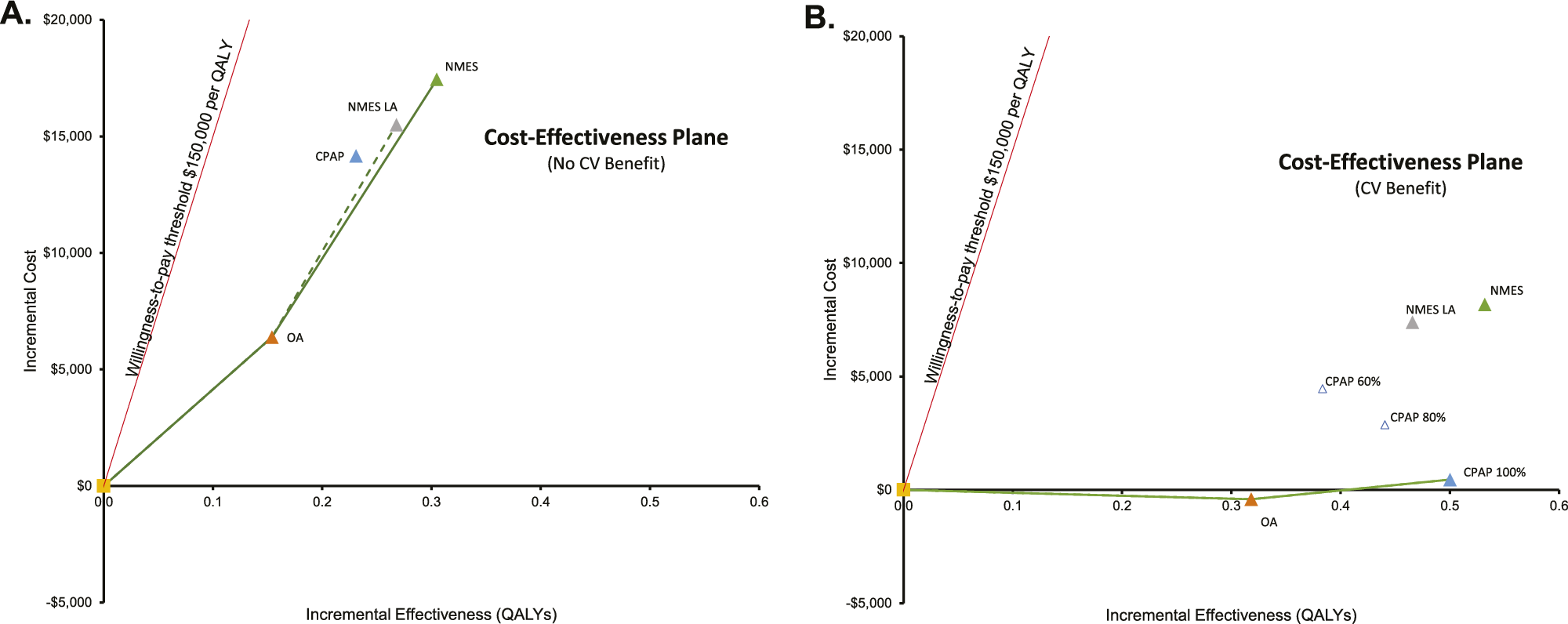
Figure 1. Lifetime cost-effectiveness estimates for NMES, OA, and CPAP versus no treatment, base case (A) and exploratory analysis with consideration of potential therapy-associated cardiovascular benefit (B). CPAP: continuous positive airway pressure; NMES: neuromuscular electrical stimulation; OA: oral appliance; LA: low adherence assumption for NMES, 65 percent long-term; CPAP 100/80/60 percent: Cardiovascular risk reduction with CPAP 100/ 80/60 percent, respectively. Interpretation of figures: On the x-axis, incremental QALYs of the respective intervention versus no treatment are shown, on the y-axis incremental lifetime costs of each therapy compared to No treatment. The green lines denote the “efficient frontier” that includes all interventions not dominated by the others. In Figure A, NMES is the preferred option, as it is cost-effective versus the next-best option in both the standard and low-adherence scenario. In Figure B, NMES is not cost-effective relative to CPAP, if CPAP nightly use is 100 percent, However, NMES would be cost-effective if CPAP nightly use was only 80 or 60 percent.
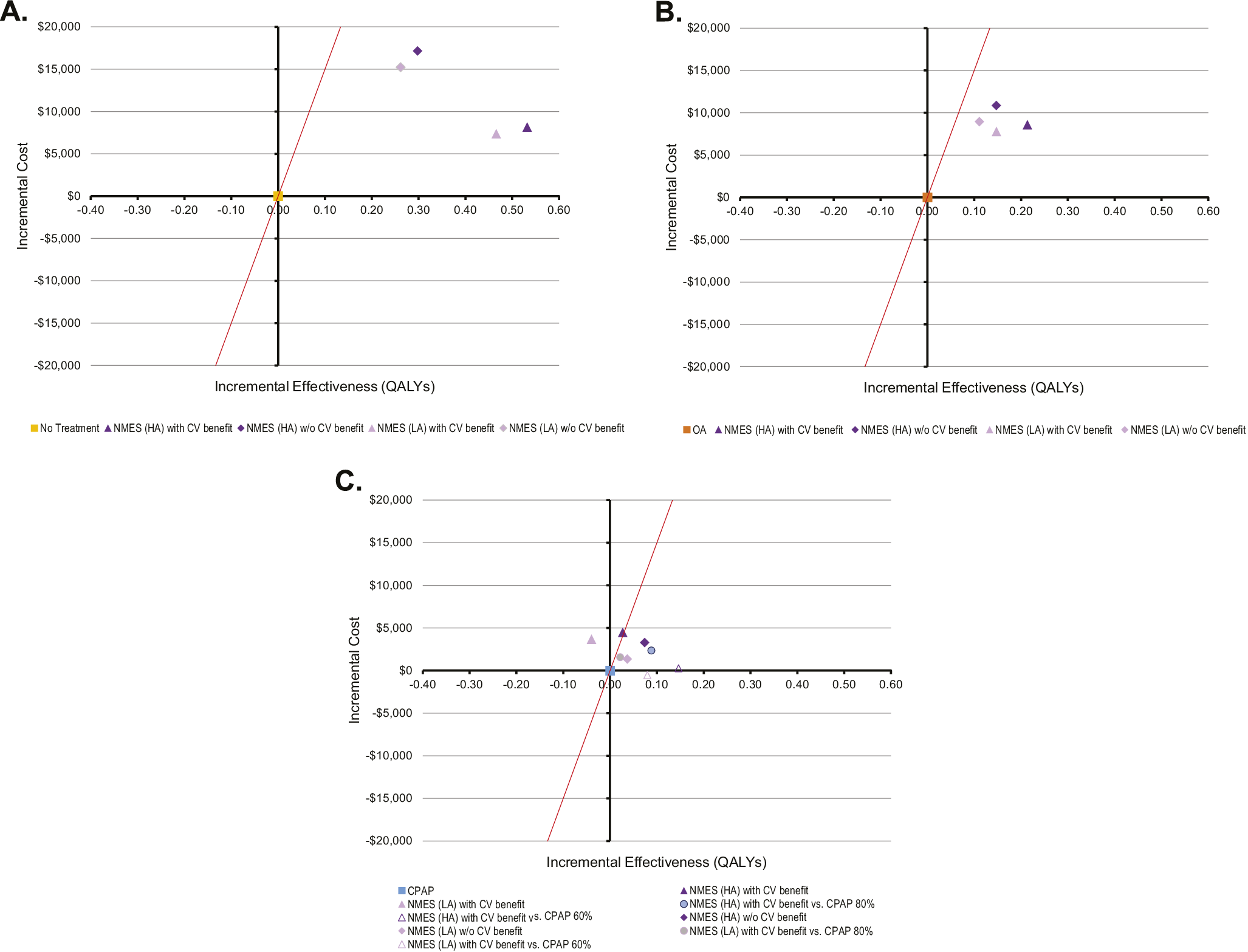
Figure 2. Lifetime cost-effectiveness estimates for NMES versus no treatment (A), NMES versus OA (B), and NMES versus CPAP (C). CPAP: continuous positive airway pressure; NMES: neuromuscular electrical stimulation; OA: oral appliance; LA: low adherence assumption for NMES, 65percent long-term; CPAP 100/80/60 percent: Cardiovascular risk reduction with CPAP 100/ 80/60 percent, respectively. Interpretation of figures: On the x-axis, incremental QALYs of the respective intervention versus no treatment are shown, on the y-axis incremental lifetime costs of each therapy compared to no treatment. The red line denotes the willingness-to-pay (WTP) threshold of USD150,000 per QALY. Scenarios are cost-effective versus no treatment as long as they lie to the right of the WTP line.
Where potential CV benefit was considered, NMES-associated QALY gains versus no treatment were larger (0.54 and 0.47 QALYs for base case and NMES low adherence scenario), and NMES cost-effectiveness versus no treatment was more favorable (Figures 1B and 2A). Compared to CPAP, incremental QALY gains with NMES were either positive or negative, depending on CPAP CV risk reduction effectiveness, resulting in a range of scenarios that rendered NMES either cost-effective or not cost-effective versus CPAP (Figure 2C). Compared to OA, NMES was found cost-effective in both the base case and the CV benefit scenario (Figure 2B).
For the adherence scenarios explored in two-way sensitivity analyses, only NMES or CPAP were the preferred strategies for all explored combinations of therapy adherence. An older cohort age of 65 marginally increased the favorability of CPAP (Figure 3A). At the same time, reduced CPAP CV effectiveness expected from only partial use of CPAP therapy during the night increased the favorability of NMES, when CV benefit was considered (Figure 3B).
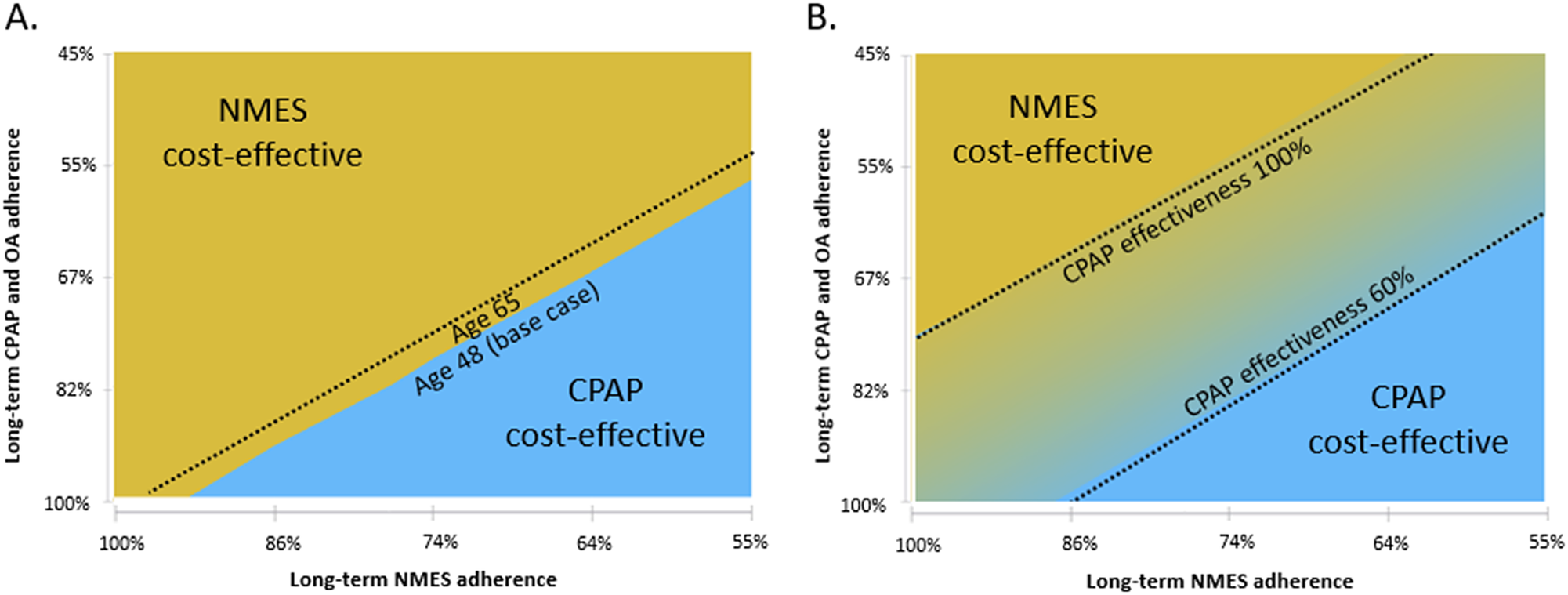
Figure 3. Two-way sensitivity analysis of the effect of variation in long-term CPAP/OA adherence versus NMES adherence on cost-effectiveness. Male cohort, no cardiovascular benefit scenario (A) and male cohort, cardiovascular benefit scenario (B). CPAP: continuous positive airway pressure; NMES: neuromuscular electrical stimulation; OA: oral appliance. Interpretation: The graphs show which therapy is preferred (at considered willingness-to-pay threshold of USD150,000 per QALY gained) for various combinations of long-term therapy adherence for NMES (x-axis) and CPAP and OA (y-axis, varied concurrently for both CPAP and OA).
Discussion
In this simulation, a comprehensive analysis framework for the evaluation of therapy options for mild OSA was presented. NMES, as a novel treatment approach not relying on nightly therapy use––and thus meeting an unmet clinical need––was found to be cost-effective versus no treatment and or the use of OA. Compared to CPAP, NMES provided improved outcomes in most analyses, and was found cost-effective versus CPAP in several but not all scenarios. Long-term adherence assumptions, CPAP therapy compliance during the night and cost assumptions were identified as key drivers determining the cost-effectiveness of NMES versus CPAP. Importantly, in the absence of negotiated allowable amounts, the current analysis relied on present manufacturer-suggested retail prices for the NMES device and disposables. A sensitivity analysis demonstrated cost savings versus CPAP when NMES reimbursement was at or below 75 percent of the base case assumption. Actual long-term prices might be lower. Thus, the current analysis findings are likely very conservative and might therefore underestimate the true long-term cost-effectiveness of NMES.
Only three prior studies have investigated the cost-effectiveness of treatments for mild OSA (46;48;49). A study from France assessed the cost-effectiveness of treatments for mild-to-moderate OSA. In that study, analyses were conducted separately for a low CV risk cohort and for a high CV risk cohort that was 56 years of age. The authors of that study reported a QALY gain of CPAP versus no treatment of 0.187 in mild OSA patients at low CV risk, closely in line with the QALY gains of 0.134 and 0.231 projected in this study for cohorts 48 and 65 years of age (Reference Poullié, Cognet and Gauthier48). McDaid et al. (Reference McDaid, Griffin and Weatherly46) in a UK health technology assessment conducted in the early 2000s, evaluated the cost-effectiveness of CPAP versus no treatment. In a scenario analysis of mild OSA patients 50 years of age, their study (“the York model”) found a lifetime gain of 0.13 QALYs, somewhat lower than the 0.20 QALYs projected in the current study for the slightly younger cohort aged 48 years. Finally, Sharples et al, in an economic analysis of the TOMADO trial that also included a model-based assessment of CPAP, projected a QALY gain of 0.304 for CPAP versus no treatment in mild-to-moderate OSA, and again found very similar QALYs for OA and CPAP, with an incremental gain of 0.02 QALYs in favor of CPAP (Reference Sharples, Glover and Clutterbuck-James49).
As is well known and confirmed herein, therapy adherence is a key driver of the cost-effectiveness of OSA treatments. This applies particularly in less severe OSA, as evidenced by the study by Jacobsen et al. that found almost half of mild OSA patients had stopped using CPAP within 3 years (Reference Jacobsen, Eriksen and Hansen7). An equal challenge appears to apply for OA, as evidenced by a review study that pooled data from 21 studies, and found OA compliance between 56 and 68 percent at an average follow-up of 33 months (Reference Hoffstein50). Importantly, even in adherent patients, insufficient nightly therapy compliance might reduce therapy effectiveness, as has been widely discussed and triggered the development of metrics such as the “effective AHI” (Reference Boyd, Upender and Walters51). The current analysis explored these scenarios through consideration of CPAP-associated CV risk reduction effectiveness of only 60 or 80 percent, as opposed to 100 percent assumed in the base case.
NMES treatment with the studied stimulation device eliminates the need for therapy during sleep time, and thus overcomes the challenge of nightly therapy compliance. Although patients need to be compliant with device use during the day for defined periods of time, there are extensive periods where patients achieve clinical benefit without any device interaction or compliance, suggesting that sustained outcomes might be achieved at a lower adherence burden compared to CPAP or OA. Additional data about the multiyear adherence and the resulting sustainable outcomes of NMES in real-world practice will be helpful to confirm the current study’s assumptions. However, it is likely that this characteristic of NMES treatment leads to additional patient benefit that is not fully captured in the current analysis.
The current study builds on a body of evidence about the implications of treated versus untreated mild OSA that is still emerging, with evidence and insight more limited compared to moderate-to-severe OSA. There is increasing recognition that mild OSA should be treated to improve quality of life and, in some patients, particularly those with CV comorbidities, might also play a role in reducing overall CV risks. The recent MERGE trial reported that three months of treatment with CPAP for mild OSA led to statistically significant improvements in the SF-36 Vitality metric by 10 points (95 percent CI 7.2–12.8) (Reference Wimms, Kelly and Turnbull33). These findings extended in subset analysis to very mild OSA patients with improvements in vitality and sleepiness metrics (Reference Kelly, Wimms and Turnbull52). At the same time, the relation between mild OSA and CV events remains controversial. Although a meta-analysis of clinical trials found no significant association between CPAP utilization and some CV events (Reference Yu, Zhou and McEvoy53), other meta-analyses have found a modest, but consistent blood pressure reduction of 2 mmHg from the utilization of CPAP (Reference Torres, Sánchez-de-la-Torre and Barbé54), which would be anticipated to translate to a reasonable reduction in CV events (Reference Hardy, Loehr and Butler55). Provided the uncertainties surrounding the CV benefit of treating mild OSA, the base case analysis in this study considered no treatment-associated CV benefit.
This study has several limitations. First, although an emerging body of clinical evidence is available for all three studied interventions, data are still limited in the mild OSA indication. Further, evidence on potential cardiovascular risk reduction in mild OSA is uncertain. The study is thus exploratory in nature rather than definitive. Comprehensive scenario and sensitivity analyses were conducted to provide insight of the effect or remaining parameter uncertainty. Second, where available, Medicare reimbursement was used as a proxy for true cost. Private payer reimbursement might differ, which to a certain extent might affect the cost-effectiveness results. However, such difference in private payer cost might be expected to apply to all therapies. Third, as outlined earlier, current cost assumptions of NMES might be significantly higher than what Medicare will ultimately pay for this intervention once allowable amounts have been set. The current analysis might therefore underestimate the long-term cost-effectiveness of NMES. Fourth, this analysis was exploratory in nature. Future, more definitive studies will benefit from more granular modeling of uncertainty and presentation of probabilistic sensitivity analyses. These were beyond the scope of the current paper. Finally, the analysis considered no sequential scenarios of treatment, for example, a patient who has failed CPAP therapy then advancing to OA or NMES as a subsequent treatment. However, insights from the current analysis might help to at least theoretically estimate the health-economic value proposition of such sequential treatments.
In conclusion, NMES––a noninvasive daytime treatment whose clinical effect is independent of night-time usage––might be a cost-effective treatment option for patients with mild OSA and may be preferred over CPAP or OA depending on adherence and cost assumptions.
Supplementary material
The supplementary material for this article can be found at http://doi.org/10.1017/S0266462323000272.
Acknowledgements
JBP had full access to all of the data in the study and takes responsibility for the integrity of the data and the accuracy of the data analysis. The sponsor provided current list prices for NMES therapy and was offered review of the manuscript for accuracy of technical and regulatory details of NMES.
Author contribution
S.L., K.N.C., A.M.G., N.M.P., and J.B.P. contributed substantially to the study design, data analysis and interpretation, and the writing of the manuscript.
Funding statement
This study was partially funded by Signifier Medical Technologies Ltd. (London, UK), manufacturer of the eXciteOSA neuromuscular electrical stimulation device studied in current analysis.
Competing interest
The authors declare none.
Financial disclosure
Wing Tech Inc. (Drs. Pietzsch, Cao, Liu, and Ms. Garner) provided health-economic consulting services to Signifier Medical Technologies Ltd. (London, UK), the manufacturer of eXciteOSA neuromuscular electrical stimulation device. Dr. Punjabi is supported by NIH grants HL11716 and HL146709. In addition, he has received grant and material support from Philips-Respironics and Resmed unrelated to this work.