Introduction
The past decade has seen a growing volume of scholarship focused on climate change and societal responses to climate change in the ancient Mediterranean region, partly fuelled by our modern-day concerns about global warming. Regarding Greece, a substantial body of new palaeoclimatological proxy data (i.e. an indirect indicator of the nature of the climate at any given time) have also been published in the past 10 years and offer new opportunities for integrated perspectives on climate and societal responses in the ancient Greek world.
The aim of the current review is to highlight recent debates on climate and society in the ancient Greek world and to consider how we can move forward within the framework of integrated archaeological and palaeoenvironmental research. The paper will provide an overview of new proxy archives from Greece, published in the last 10 years, which provide data at a sufficient resolution for periods relevant to archaeologists and historians (corresponding to the Middle and Late Holocene in geological time scales encompassing approximately the past 8,000 years). The review will also present a more focused discussion on the state of the available palaeoclimatological proxy data for the first millennium BC, including the Archaic, Classical, and Hellenistic periods, i.e. periods that will be of specific interest to the readers of Archaeological Reports but which have not been extensively discussed within previous research on human–environment interactions in archaeology.
Approaches to climate, environment, and society
Until recently, studies of climate and society in Greece and the eastern Mediterranean were focused primarily on the impact of climate change on societal collapse. Such research has generally been focused on the breakdown of societal organization and settlement structures in light of catastrophic climate events, which prompted periods of extraordinarily arid conditions in the Late Bronze Age (for example, Cullen et al. Reference Cullen, deMenocal, Hemming, Hemming, Brown, Guilderson and Sirocko2000; Middleton Reference Middleton2010; Kaniewski, Guiot and Van Campo Reference Kaniewski, Guiot and Van Campo2015; Kaniewski and Van Campo Reference Kaniewski, Van Campo and Weiss2017; Weiss Reference Weiss and Weiss2017; see also the review in Weiberg and Finné Reference Weiberg and Finné2022). In a Greek context, research has focused on the possible impact of the climate in the destruction of the Mycenaean palatial system, at the time of the so-called ‘3.2k event’ (referring to 3200 BP in scientific dating, i.e. 1250 BC), which appears as a pronounced dry sequence in some (but not all) eastern Mediterranean palaeoenvironmental archives (Middleton, Reference Middleton2010; Drake Reference Drake2012; Cline Reference Cline2014; Knapp and Manning Reference Knapp and Manning2016; Finné et al. Reference Finné, Holmgren, Shen, Hu, Boyd and Stocker2017; Vidal-Cordasco and Nuevo-López Reference Vidal-Cordasco and Nuevo-López2021; see also discussion in Weiberg and Finné Reference Weiberg and Finné2018 with references). The focus on the interplay between climate change and collapse has at times been problematic, since it has tended to privilege climate as the primary cause behind societal breakdown without properly focusing on the nuances of socioeconomic structures that would have been impacted by climate change. Instead, the synchronicity of arid conditions and collapse has often been used as an indicator of direct climate impact. However, some of the research focusing on aspects of the Late Bronze Age collapse has been keen to stress that climate is part of a longer process prompting societal change. Brandon Drake (Reference Drake2012: 1,866), for example, refers to climate trajectories in the late second millennium BC as a ‘gear shift in Mediterranean climate’ that would not have constituted an ‘event’ but rather a process continuously putting stress on the system, necessitating analyses that also incorporate earlier climate trajectories and the interplay between longer processes and more rapid climate events. These issues have been treated in several studies carried out within the framework of the Domesticated Landscapes of the Peloponnese project (DoLP) at Uppsala University, Sweden. Studies of the later Bronze Age (LHIA–IIIC) carried out within the project have shown the importance of looking at the full span of the Late Helladic climate variability before the disappearance of the Mycenaean Palatial systems (Weiberg and Finné Reference Weiberg and Finné2018; Reference Weiberg, Finné, Erdkamp, Manning and Verboven2021). This research has shown that the build-up of socioeconomic structures and their expansion in periods of more favourable climatic conditions created agricultural vulnerabilities in the face of ensuing arid conditions, specifically in societies with limited adaptive capacity (Weiberg and Finné Reference Weiberg, Finné, Erdkamp, Manning and Verboven2021). The focus on long- and medium-term developments, societal resilience, and climate variability has provided a more nuanced discussion on the impact of climate on Late Bronze Age societies going beyond the synchronicity between climate events and collapse. The recent study of Riia Timonen and Ann Brysbaert (Reference Timonen, Brysbaert, Erdkamp, Manning and Verboven2021), published in a collection of papers stemming from a Brussels conference on climate and ancient societies in Europe and the Near East (Erdkamp, Manning and Verboven Reference Erdkamp, Manning and Verboven2021), also offers a close analysis of how drier climate may have impacted specific aspects of subsistence, agricultural production, and monumental building programmes in the Argive plain in the Mycenaean period. While the study does not adopt the same long-term framework as the research by the DoLP group, it does however highlight the importance of examining how changes in the timing and amount of precipitation over time could have impacted specific features of the Mycenaean regional economies.
Going beyond the Late Bronze Age, scholarship on climate change and socio-environmental dynamics in the broader Mediterranean has in recent years been largely focused on different periods of Late Antiquity (see for example Haldon et al. Reference Haldon, Roberts, Izdebski, Fleitmann, McCormick, Cassis, Doonan, Eastwood, Elton and Ladstätter2014; Reference Haldon, Mordechai, Newfield, Chase, Izdebski, Guzowski, Labuhn and Roberts2018c; Haldon and Rosen Reference Haldon and Rosen2018; Izdebski and Mulryan Reference Izdebski and Mulryan2019). Kyle Harper’s book on climate change in the later Roman Empire (Reference Harper2017) has prompted much critique and debate on the possible impact of climate on societal transformations in this period (Erdkamp Reference Erdkamp2019; Haldon et al. Reference Haldon, Elton, Huebner, Izdebski, Mordechai and Newfield2018a; Reference Haldon, Elton, Huebner, Izdebski, Mordechai and Newfield2018b). Discussions on climate change and socio-environmental trajectories in the Greek world during the historical periods have, however, been much more limited, particularly in the context of the Archaic, Classical, and Hellenistic eras (as has also been discussed in Post Reference Post2022a; Weiberg and Finné Reference Weiberg and Finné2022). Multidisciplinary research on Egypt and the impact of volcanic eruptions on the Nile summer floods, disruptions in agricultural production, and societal responses during the Hellenistic period has been carried out by Joseph Manning and colleagues, utilizing Arctic ice core data (Manning et al. Reference Manning, Ludlow, Stine, Boos, Sigl and Marlon2017; Ludlow and Manning Reference Ludlow, Manning, Erdkamp, Manning and Verboven2021). This, however, represents a very distinct climatic system and the proxies used are not immediately relevant to other areas of the ancient Greek world.
Some interest in climate variability in Aegean Greece during the historical periods has been shown by historians working on ancient Greek economies. Alain Bresson, for example, offered a general discussion in his 2014 paper on climate and society in Greco-Roman antiquity, even though the palaeoclimatic proxy data that he used was of a more general and non-local nature (Bresson Reference Bresson and de Callataÿ2014). More recently Ruben Post utilized available proxy records from the northern Peloponnese to explore the ban on grain exports enforced by the Achaian league between ca 191 and 188 BC, arguing that increasingly dry conditions as inferred from the proxy data could have played into the restrictions imposed by the federal community (Post Reference Post2022b). In terms of research focused on the Greek archaeological record, members of the DoLP research group have further provided new comparative analyses of land use and climate in the Classical, Hellenistic, and Roman periods, particularly focused on the northeastern Peloponnese (Bonnier and Finné Reference Bonnier and Finné2020; Reference Bonnier, Finné, Erdkamp, Manning and Verboven2021). The approach utilized in these two studies is focused specifically on agricultural economies and on how the spatial extent of land use would have shifted in light of changes in hydroclimate, as is evident from archaeological survey data (ID13553, ID16793, ID16795, ID16682, ID10460, ID16448) and palaeoclimatic proxy records from speleothems. Unlike much of the research carried out for the Late Bronze Age and Late Antiquity, the interest in these periods has not been immediately tied to questions of societal breakdowns and fragmentation but rather how the trajectories provided by palaeoclimatic reconstructions can be connected with shifts in agricultural land use in both humid and drier periods. These studies, nevertheless, also discuss the interplay between sociopolitical changes and environmental dynamics in the Peloponnese, stressing the importance of integrating the environmental data with broader political and economic factors without privileging climate change as a primary cause.
Much recent work has also taken broader diachronic perspectives on human responses to climate variability and environmental changes over longer periods in Greece and the Aegean. Such syntheses have been produced by scholars working within both archaeology and environmental sciences, highlighting the importance of understanding climate variability and socio-environmental developments both in light of negative as well as beneficial climate change, primarily in terms of dry or humid conditions (Weiberg et al. Reference Weiberg, Unkel, Kouli, Holmgren, Avramidis, Bonnier, Dibble, Finné, Izdebski, Katrantsiotis, Stocker, Andwinge, Baika, Boyd and Heymann2016; Reference Weiberg, Bevan, Kouli, Katsianis, Woodbridge, Bonnier, Engel, Finné, Fyfe, Maniatis, Palmisano, Panajiotidis, Roberts and Shennan2019; Weiberg, Bonnier and Finné Reference Weiberg, Bonnier and Finné2021). The work carried out by the DoLP group, which uses GIS-based spatial analyses of survey data and palaeoclimatic sequences from cave records, displays staggering synchronicity between archaeological periods of land use expansion and above-average wet conditions in most, but not all, periods (a notable exception is the MHIII-LHI time frame). This type of comparison was possible through the grouping of palaeoclimatic proxy data according to the relative archaeological periods using the proportion of data points displaying above-average wet conditions from Peloponnesian speleothems (Bonnier, Finné and Weiberg Reference Bonnier, Finné and Weiberg2019; Weiberg, Bonnier, and Finné Reference Weiberg, Bonnier and Finné2021; Fig. 7.1). The study thus shows the importance of adapting chronological resolution to facilitate comparison between proxy data and the archaeological record (Weiberg and Finné Reference Weiberg and Finné2022). This research also highlights how humid climate conditions may have helped to push land use and cultivation in areas of the landscape with thinner soil cover in different periods (Bonnier and Finné Reference Bonnier, Finné, Erdkamp, Manning and Verboven2021; Weiberg, Bonnier and Finné Reference Weiberg, Bonnier and Finné2021; Fig. 7.2). Land use expansion under wetter conditions may, in turn, have increased the vulnerability of farmers operating in more marginal areas in subsequent dry periods, particularly in naturally drier areas of the Greek mainland and the Aegean such as Attica and the northeastern Peloponnese. These analyses of climate and land-use patterns should ideally also be paralleled with future research adopting more site-specific analyses of archaeological material. A recent study by Flint Dibble and Martin Finné (Reference Dibble and Finné2021) focusing on excavated faunal assemblages highlights, for example, how climate can be understood as a possible factor of changing foodways and agricultural strategies in the transition from the Bronze Age to the Early Iron Age in Greece. Such research emphasizes the importance of integrating different forms of archaeological data with palaeoenvironmental sequences to track the various features of society that may have been impacted by climate changes over time.
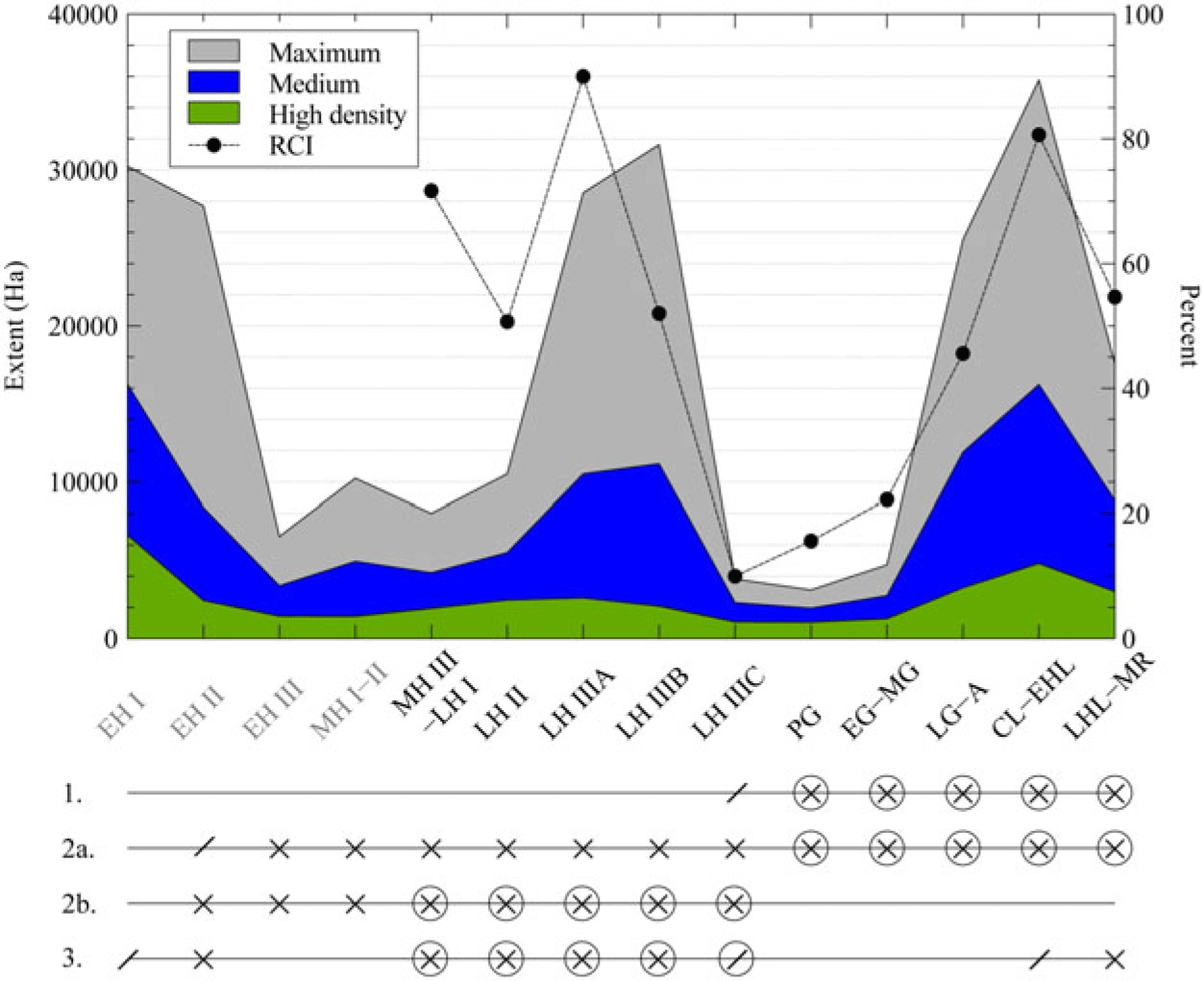
7.1. Land use and climate trends in the northeastern Peloponnese. Changes in the maximum extent, medium extent, and high-density extent of land use as derived from GIS-based kernel density analysis are shown in hectare values together with the relative climate index (RCI). The RCI is based on the number of above-average humid data points in the Kapsia, Alepotrypa, and Mavri Trypa datasets according to the relative archaeological chronology. A higher RCI value is suggestive of more humid climate conditions (Weiberg, Bonnier and Finné Reference Weiberg, Bonnier and Finné2021: fig. 2).

7.2. Land use and climate trends in the northeastern Peloponnese. Changes in the proportion of Slope Class 2 in maximum extent of the extent of possible land uses (EPLUs) as derived from GIS-based kernel density analysis together with the RCI. Slope Class 2 is composed of sloping ground that is susceptible to erosion when cultivated without terracing. The RCI is based on the number of above-average humid data points in the Kapsia, Alepotrypa, and Mavri Trypa datasets according to the relative archaeological chronology. A higher RCI value is suggestive of more humid climate conditions (after Weiberg, Bonnier and Finné Reference Weiberg, Bonnier and Finné2021: fig. 3).
In general, much of this recent research has been thoroughly interdisciplinary, involving scholars from different fields. It has also prompted the development of new comparative methods and integrated approaches. The methodological developments in turn provide possibilities for new ways of integrating and thinking about causation and socio-environmental dynamics. The work thus highlights the importance of achieving consilience between scholars from various disciplines working together in both project design and research, providing methodological balances (see Izdebski et al. Reference Izdebski, Holmgren, Weiberg, Stocker, Büntgen, Florenzano, Gogou, Leroy, Luterbacher, Martrat, Masi, Mercuri, Montagna, Sadori, Schneider, Sicre, Triantaphyllou and Xoplaki2016). In particular, all researchers of different disciplinary backgrounds need to be aware of the limitations and margins of error present in the palaeoenvironmental proxy data as well as the complexities inherent in using historical evidence and archaeology to examine societal dynamics in light of environmental change.
Proxy data: new archives and new methodological approaches
The past decade has seen a substantial increase in the amount of palaeoclimatic proxy data available from Greece. Given the scarcity of ancient written testimony on weather and climate, palaeoclimatological proxies offer essential evidence for addressing questions on human–environment interactions and possible climate impact on societies in the ancient Greek world (Finné et al. Reference Finné, Holmgren, Sundqvist, Weiberg and Lindblom2011; Erdkamp Reference Erdkamp, Erdkamp, Manning and Verboven2021). The use of proxy data is, however, complicated by aspects of chronological resolution and spatial coverage as well as differences in terms of what the signals indicate with regard to climate, for example, changes in temperature and/or precipitation (Weiberg et al. Reference Weiberg, Unkel, Kouli, Holmgren, Avramidis, Bonnier, Dibble, Finné, Izdebski, Katrantsiotis, Stocker, Andwinge, Baika, Boyd and Heymann2016; Finné and Weiberg Reference Finné, Weiberg, Ekblom, Isendahl and Lindholm2018; Finné et al. Reference Finné, Woodbridge, Labuhn and Roberts2019). Environmental and geographical factors further limit the types of records that are available. In many parts of temperate Europe, tree ring data offer some of the best high-resolution climate proxies but such data is limited for Greece and the data series that do exist from northern Greece only cover later periods, spanning the Middle Ages from AD 1089 onwards (Griggs et al. Reference Griggs, DeGaetano, Kuniholm and Newton2007; Klippel et al. Reference Klippel, Krusic, Brandes, Hartl, Belmecheri, Dienst and Esper2018; Esper et al. Reference Esper, Konter, Klippel, Krusic and Büntgen2021). Most of the recently published proxy data from Greece consist, instead, of sediment records extracted from lacustrine and marine environments as well as climate proxies derived from cave speleothems (Finné et al. Reference Finné, Holmgren, Sundqvist, Weiberg and Lindblom2011; Reference Finné, Woodbridge, Labuhn and Roberts2019; Weiberg et al. Reference Weiberg, Unkel, Kouli, Holmgren, Avramidis, Bonnier, Dibble, Finné, Izdebski, Katrantsiotis, Stocker, Andwinge, Baika, Boyd and Heymann2016).
Proxy records from lake, wetland, and marine sediments (Map 7.1)

Map 7.1. Sites referred to in text: 1. Lake Prespa; 2. Aliki; 3. Vouliagmeni; 4. Klisova lagoon; 5. Lake Dojran; 6. Etoliko lagoon; 7. Lake Trichonida; 8. Pheneos valley; 9. Kaisari valley; 10. Lake Stymphalos; 11. Asea valley; 12. Messara plain; 13. Lake Kournas; 14. Aghios Floros; 15. Lake Lerna; 16. Gialova lagoon; 17. M2; 18. Alepotrypa; 19. Mavri Trypa; 20. Kapsia; 21. Skala Marion; 22. Brauron; 23. Eleusis Bay. © BSA.
Coring in lakes and wetland areas can provide complex and multifaceted environmental archives that include palaeoclimatic proxy data as well as other signals (Weiberg et al. Reference Weiberg, Unkel, Kouli, Holmgren, Avramidis, Bonnier, Dibble, Finné, Izdebski, Katrantsiotis, Stocker, Andwinge, Baika, Boyd and Heymann2016). Palaeoclimatic signals from lake records can be inferred from changes in the hydrological balance recorded by different proxies. The ratio of total organic carbon and total nitrogen stored in sediments has, for example, been used to determine depositional conditions and can potentially be tied to palaeoclimatic changes affecting lake levels (Avramidis et al. Reference Avramidis, Geraga, Lazarova and Kontopoulos2013), even if factors other than climate may also impact the ratio. Hydrogen isotopes (δ18O) from lake sediments have also been widely used in the Mediterranean region as a climate proxy (Roberts et al. Reference Roberts, Jones, Benkaddour, Eastwood, Filippi, Frogley, Lamb, Leng, Reed, Stein, Stevens, Valero-Garcés and Zanchetta2008). In terms of recent trends within research on lake sediments and palaeoclimatology in Greece, much new work has utilized X-ray fluorescence (XRF) analysis of geochemical elements in combination with Bayesian age-depth models – in the past decade, this method of analysis has been applied on sediment cores from Lake Prespa (Aufgebauer et al. Reference Aufgebauer, Panagiotopoulos, Wagner, Schaebitz, Viehberg, Vogel, Zanchetta, Sulpizio, Leng and Damaschke2012); Lake Dojran (Francke et al. Reference Francke, Wagner, Leng and Rethemeyer2013); Etoliko lagoon (Haenssler et al. Reference Haenssler, Nadeau, Vött and Unkel2013); Kotychi lagoon (Haenssler et al. Reference Haenssler, Unkel, Dörfler and Nadeau2014); Asea valley (Unkel et al. Reference Unkel, Schimmelmann, Shriner, Forsén, Heymann and Brückner2014); Lake Stymphalos (Seguin et al. Reference Seguin, Bintliff, Grootes, Bauersachs, Dörfler, Heymann, Manning, Müller, Nadeau, Nelle, Steier, Weber, Wild, Zagana and Unkel2019); Aliki (Boiotia) (Emmanouilidis et al. Reference Emmanouilidis, Unkel, Triantaphyllou and Avramidis2020b); Lake Trichonida (Seguin et al. Reference Seguin, Avramidis, Dörfler, Emmanouilidis and Unkel2020a); Pheneos and Kaisari valleys (Seguin et al. Reference Seguin, Avramidis, Haug, Kessler, Schimmelmann and Unkel2020b); Lake Vouliagmeni (Perachora) (Emmanouilidis Reference Emmanouilidis, Katrantsiotis, Dotsika, Kokkalas, Unkel and Avramidis2022a); Klisova lagoon (Emmanouilidis et al. Reference Emmanouilidis, Panagiotopoulos, Kouli and Avramidis2022b). XRF scans provide the ratio of selected elements in extracted sediments, and some of these have been linked to precipitation and lake water evaporation. Variations in the ratio of rubidium and strontium (Rb/Sr ratio) have, for example, been used in multiple studies as proxies for chemical weathering intensity (Unkel et al. Reference Unkel, Schimmelmann, Shriner, Forsén, Heymann and Brückner2014; Emmanouilidis et al. Reference Emmanouilidis, Unkel, Seguin, Keklikoglou, Gianni and Avramidis2020a; Seguin et al. Reference Seguin, Avramidis, Dörfler, Emmanouilidis and Unkel2020a; Reference Seguin, Avramidis, Haug, Kessler, Schimmelmann and Unkel2020b). A higher ratio of Rb/Sr is suggestive of weak weathering and can be linked to increased precipitation in lake catchments while a decreasing Rb/Sr ratio has been associated with dry conditions (Unkel et al. Reference Unkel, Schimmelmann, Shriner, Forsén, Heymann and Brückner2014; Emmanouilidis et al. Reference Emmanouilidis, Unkel, Triantaphyllou and Avramidis2020b). Clay minerals, including potassium (K) and zirconium (Zr), have also been used to determine run-off patterns and erosion, which can potentially be linked to changes in hydroclimate. Carbonate proxies, such as the ratio of calcium and strontium (Ca/Sr ratio), can further provide evidence of summer evaporation and a high Ca/Sr ratio is indicative of lower lake levels (Heymann et al. Reference Heymann, Nelle, Dörfler, Zagana, Nowaczyk, Xue and Unkel2013; Unkel et al. Reference Unkel, Schimmelmann, Shriner, Forsén, Heymann and Brückner2014; Seguin et al. Reference Seguin, Avramidis, Dörfler, Emmanouilidis and Unkel2020a). Statistical approaches and, in particular, principal component analysis grouping geochemical elements derived from XRF analysis have been employed to examine variation in component groups. The groups presenting the most variation in the data (PC1) have been used in several studies as a signal related to hydroclimatic trends (Seguin et al. Reference Seguin, Avramidis, Dörfler, Emmanouilidis and Unkel2020a; Reference Seguin, Avramidis, Haug, Kessler, Schimmelmann and Unkel2020b; Emmanouilidis et al. Reference Emmanouilidis, Katrantsiotis, Dotsika, Kokkalas, Unkel and Avramidis2022a).
Recent work on sediments from Greek lake records has also been focused on new methods and analyses of biological proxies. In the past 10 years, the study of diatoms as potential climate proxies has been carried out at multiple lacustrine archives in the Greek mainland: Lake Dojran (Francke et al. Reference Francke, Wagner, Leng and Rethemeyer2013); Lake Prespa (Cvetkoska et al. Reference Cvetkoska, Levkov, Reed and Wagner2014); Aghios Floros (Katrantsiotis et al. Reference Katrantsiotis, Norström, Holmgren, Risberg and Skelton2016); Messara plain (Crete) (Ghilardi et al. Reference Ghilardi, Psomiadis, Andrieu-Ponel, Colleu, Sotiropoulos, Longo, Rossi, Amato, Gasse, Sinibaldi, Renard, Bicket, Delanghe, Demory and Fleury2018; Reference Ghilardi, Revelles, Glais, Theodorakopoulou, Theodoropoulou, Lespez, Longo, Rossi, Bellier, Benedetti and Fleury2019); Lake Kournas (Crete) (Jouffroy-Bapicot et al. Reference Jouffroy-Bapicot, Pedrotta, Debret, Field, Sulpizio, Zanchetta, Sabatier, Roberts, Tinner, Walsh and Vannière2021) and Lake Vouliagmeni (Emmanouilidis et al. Reference Emmanouilidis, Katrantsiotis, Dotsika, Kokkalas, Unkel and Avramidis2022a). Diatoms are single-celled species of algae that exist in lake environments, and different species cluster in certain hydraulic environments. The study of diatoms in cored sediments can therefore be used to provide evidence of hydrological change, especially when complemented with data series for carbon and nitrogen isotopes (Seguin et al. Reference Seguin, Avramidis, Haug, Kessler, Schimmelmann and Unkel2020b). Diatoms may, for example, be used to examine shifts in lake levels that can be indicative of precipitation and evaporation, i.e. relating both to hydroclimate and changes in temperature as well as water depth impacted by tectonics (Norström et al. Reference Norström, Katrantsiotis, Finné, Risberg, Smittenberg and Bjursäter2018).
Another interesting development in terms of Greek palaeoclimatic proxies from lake records is the analysis of carbon and hydrogen isotopes in leaf wax-derived n-alkanes from terrestrial and aquatic plants (Aghios Floros: Norström et al. Reference Norström, Katrantsiotis, Smittenberg and Kouli2017; Reference Norström, Katrantsiotis, Finné, Risberg, Smittenberg and Bjursäter2018; Gialova lagoon: Katrantsiotis et al. Reference Katrantsiotis, Kylander, Smittenberg, Yamoah, Hättestrand, Avramidis, Strandberg and Norström2018; Lake Lerna: Katrantsiotis et al. Reference Katrantsiotis, Norström, Smittenberg, Finné, Weiberg, Hättestrand, Avramidis and Wastegård2019; Tenaghi Philippon: Schemmel et al. Reference Schemmel, Niedermeyer, Koutsodendris, Pross, Fiebig and Mulch2017; Lake Dojran: Thienemann et al. Reference Thienemann, Masi, Kusch, Sadori, John, Francke, Wagner and Rethemeyer2017). N-alkanes are molecules that consist of carbon and hydrogen produced by plants either algae, aquatic macrophytes, or terrestrial. Different isotopes of hydrogen can be incorporated into the molecules and the measurable ratio between these forms of hydrogen (denoted δD) can serve as a proxy for hydroclimate change, since variations are linked to changes in the source water that is absorbed by the plants. The isotope composition of the source water is in turn affected by both precipitation and temperature (Norström et al. Reference Norström, Katrantsiotis, Finné, Risberg, Smittenberg and Bjursäter2018). At both Aghios Floros and Lerna, the proportion of δD23 from aquatic macrophytes has, for example, been used to determine shifts between humid and drier conditions (Norström et al. Reference Norström, Katrantsiotis, Finné, Risberg, Smittenberg and Bjursäter2018; Katrantsiotis et al. Reference Katrantsiotis, Norström, Smittenberg, Finné, Weiberg, Hättestrand, Avramidis and Wastegård2019). Carbon isotopes (δ13C) of terrestrial plant wax-derived alkanes have further been used at Aghios Floros to track changes in drought-adapted plant communities (Norström et al. Reference Norström, Katrantsiotis, Smittenberg and Kouli2017; Reference Norström, Katrantsiotis, Finné, Risberg, Smittenberg and Bjursäter2018).
Overall, the production of new proxy series from sedimentary sequences (from lakes and wetlands) has added new data indicating climate variability that can be of use to archaeologists working on human–environment interactions in the ancient Greek world. There are, however, issues and potential challenges that need to be kept in mind and that complicate the use of these records. Changes in the hydrological environments can be linked to shifts in precipitation and temperature, while the geochemical composition of sediments can also be linked to human activities in the lake catchments (Seguin et al. Reference Seguin, Avramidis, Haug, Kessler, Schimmelmann and Unkel2020b). Clearance of vegetation in connection with farming may, for example, increase surface run-off and accelerate erosion in the catchments, which, in turn, may affect lake levels. Anthropogenically-forced erosion can thus provide similar signals in the lake records to changes in hydroclimate (see discussion in Seguin et al. Reference Seguin, Avramidis, Haug, Kessler, Schimmelmann and Unkel2020b). The recent analysis of the isotopic composition of the n-alkanes may in this sense be a way to overcome some of these problems as the data reflects the isotopic composition carried through the source water of the plants producing the leaf waxes. In particular, the δD23 signal can be useful as it is attributed to submerged or floating macrophytes and can therefore not be tied to run-off derived from the catchments (Katrantsiotis et al. Reference Katrantsiotis, Norström, Smittenberg, Finné, Weiberg, Hättestrand, Avramidis and Wastegård2019). However, a problem linked to the use of the isotopic composition of the n-alkanes from macrophytes is that in Greece the source water can come from karstic springs, as in the case of Lake Lerna. The input of water from karstic springs makes the interpretation more complex, since the origin in both time and space of the source water can be complex. Lake records should, nonetheless, be seen as reflecting primarily local environmental conditions that should be analysed in connection with human impact on the surrounding landscape. Archaeology can therefore play an important part in deducing the level of anthropogenic impact that may disturb the palaeoclimatic signals in the lake proxy records.
In contrast to lake records discussed above, cored sediments from marine environments contain proxy data that offer more regional signals. Methods used in the analysis of sediment cores from marine environments have included studies of stable isotopes from sediments and lipid biomarkers as well as studies of biological proxies, such as coccolithophores, foraminifera, ostracods, and dinoflagellates, to examine changes in seasonal sea surface temperature (Kuhnt et al. Reference Kuhnt, Schmiedl, Ehrmann, Hamann and Hemleben2007; Chen, Zonneveld and Versteegh Reference Chen, Zonneveld and Versteegh2011; Gogou et al. Reference Gogou, Triantaphyllou, Xoplaki, Izdebski, Parinos, Dimiza, Bouloubassi, Luterbacher, Kouli and Martrat2016). The amount of recently published high-resolution data from Greek waters is, however, limited. A single core providing high-resolution palaeoclimatic data from the northern Aegean has been published within the past decade (multicore M2), but this record does not provide any data before the first millennium AD (Gogou et al. Reference Gogou, Triantaphyllou, Xoplaki, Izdebski, Parinos, Dimiza, Bouloubassi, Luterbacher, Kouli and Martrat2016). Several marine records published before the past decade provide evidence that span periods of antiquity (Geraga et al. Reference Geraga, Tsaila-Monopolis, Ioakim, Papatheodorou and Ferentinos2000; Reference Geraga, Tsaila-Monopolis, Ioakim, Papatheodorou and Ferentinos2005; Reference Geraga, Ioakim, Lykousis, Tsaila-Monopolis and Mylona2010; Rohling et al. Reference Rohling, Mayewski, Abu-Zied, Casford and Hayes2002; Kuhnt et al. Reference Kuhnt, Schmiedl, Ehrmann, Hamann and Hemleben2007), though some of the data is at comparatively low temporal resolution, making the integration with archaeological datasets difficult.
Pollen records
A biological proxy that has been extensively sampled from both lake and marine sediment records in Greece is fossilized pollen grains, a proxy which can be used to reconstruct vegetation changes over time (Weiberg et al. Reference Weiberg, Unkel, Kouli, Holmgren, Avramidis, Bonnier, Dibble, Finné, Izdebski, Katrantsiotis, Stocker, Andwinge, Baika, Boyd and Heymann2016). Changes in the proportion of pollen have, in past research, been used as a proxy for climate changes relating both to temperature and precipitation in longer time series. Specifically, arboreal pollen has been used to examine the impact of climatic changes on regional vegetation, since specific arboreal taxa such as pinus and abies thrive under humid conditions (Aufgebauer et al. Reference Aufgebauer, Panagiotopoulos, Wagner, Schaebitz, Viehberg, Vogel, Zanchetta, Sulpizio, Leng and Damaschke2012). A decrease in the overall proportion of arboreal pollen and an increase in the proportion of shrubland vegetation has thus been linked to more arid conditions, particularly in the Early Holocene as well as earlier periods (Panagiotopoulos et al. Reference Panagiotopoulos, Aufgebauer, Schäbitz and Wagner2013; Pross et al. Reference Pross, Koutsodendris, Christanis, Fischer, Fletcher, Hardiman, Kalaitzidis, Knipping, Kotthoff, Milner, Müller, Schmiedl, Siavalas, Tzedakis and Wulf2015). There are, however, significant problems with using pollen as a climate signal for the Middle and Late Holocene in Greece (as well as in the Mediterranean at large) due to the anthropogenic impact on vegetation during these periods (Calò et al. Reference Calò, Henne, Curry, Magny, Vescovi, La Mantia, Pasta, Vannière and Tinner2012; Kouli Reference Kouli2012; Kyrikou et al. Reference Kyrikou, Kouli, Triantaphyllou, Dimiza, Gogou, Panagiotopoulos, Anagnostou and Karageorgis2020). Woodland clearance due to expanding agricultural land use will, for example, have severely impacted the potential climatic signal of arboreal taxa. Previous comparisons between archaeological site data and pollen records have demonstrated that deforestation and a reduction in the proportion of arboreal taxa occur in periods when we also observe an expansion of site numbers and heavy anthropogenic impact on the landscape (Weiberg et al. Reference Weiberg, Unkel, Kouli, Holmgren, Avramidis, Bonnier, Dibble, Finné, Izdebski, Katrantsiotis, Stocker, Andwinge, Baika, Boyd and Heymann2016; Reference Weiberg, Bevan, Kouli, Katsianis, Woodbridge, Bonnier, Engel, Finné, Fyfe, Maniatis, Palmisano, Panajiotidis, Roberts and Shennan2019). Contraction of land use can further result in the regeneration of woodlands also under comparatively dry conditions. Pollen data can therefore not be used as a reliable palaeoclimatic proxy from at least the Late Neolithic/Early Bronze Age onwards in Greece.
Pollen records, nevertheless, provide crucial evidence for changes in landscape and shifts in agricultural strategies that can be used to examine societal responses to climate change, as inferred from other proxies. The variability of different taxa provides indications of vegetation changes that may be linked to changes in land-use patterns, cultivation strategies and economic practices (Weiberg et al. Reference Weiberg, Bevan, Kouli, Katsianis, Woodbridge, Bonnier, Engel, Finné, Fyfe, Maniatis, Palmisano, Panajiotidis, Roberts and Shennan2019; Weiberg, Bonnier and Finné Reference Weiberg, Bonnier and Finné2021); but there are issues connected with the use of pollen data for archaeological research that needs to be kept in mind. Chronology is a key factor in how we use and compare pollen data together with archaeological evidence and there are often dating issues inherent in the pollen data. As in the case of other proxy records, dating issues are important to consider, especially in connection with older records, while new records often have more robust age-depth models based on multiple C14 dates (Finné and Weiberg Reference Finné, Weiberg, Ekblom, Isendahl and Lindholm2018).
The spatial context of pollen archives is also important to consider. Different taxa provide different spatial signals. Both abies and pinus are often present as the largest proportion in records and generally originate from a much broader area compared to cultivars such as cereals, which enter the records primarily from the local area. The spatial context of the data cannot, therefore, be solely equated with the catchment area of, for example, the lake archives providing the pollen sequences (even if this is the case for some taxa). The location of the archives should also be considered when using different datasets. Pollen grains are primarily preserved in lake, wetland, or within marine sediments, meaning that the recovery of data is largely restricted to very specific areas of the landscape that may not be situated close to where we have recorded archaeological data. Pollen records from southern Greece are currently constituted by archives primarily in lowland areas, usually coastal environments, while in northern Greece the available pollen records generally come from inland locations (Weiberg et al. Reference Weiberg, Unkel, Kouli, Holmgren, Avramidis, Bonnier, Dibble, Finné, Izdebski, Katrantsiotis, Stocker, Andwinge, Baika, Boyd and Heymann2016; Reference Weiberg, Bevan, Kouli, Katsianis, Woodbridge, Bonnier, Engel, Finné, Fyfe, Maniatis, Palmisano, Panajiotidis, Roberts and Shennan2019; Izdebski et al. Reference Izdebski, Słoczyński, Bonnier, Koloch and Kouli2020). The environmental context of the different archives should therefore be kept in mind when looking at differences in land cover trajectories between different areas of Greece (see discussion in Weiberg et al. Reference Weiberg, Bevan, Kouli, Katsianis, Woodbridge, Bonnier, Engel, Finné, Fyfe, Maniatis, Palmisano, Panajiotidis, Roberts and Shennan2019).
Recent research has also utilized statistical methods for looking beyond individual records and has instead attempted to synthesize data to examine diachronic regional trends. Weiberg and colleagues (Reference Weiberg, Bevan, Kouli, Katsianis, Woodbridge, Bonnier, Engel, Finné, Fyfe, Maniatis, Palmisano, Panajiotidis, Roberts and Shennan2019), for example, have used cluster analysis of multiple archives in both southern and northern Greece in 200-year time windows (for a description of the methods, see also Woodbridge, Roberts and Fyfe Reference Woodbridge, Roberts and Fyfe2018). The broader trends in terms of vegetation cover resulting from this study can also be linked with regional economic developments and land use pressure in both prehistory and the historical periods. Panel data models based on interpolations of values from multiple pollen records from southern Greece have recently also been used to investigate changes in cultivation patterns and links between land use and trade in ancient Greece (Izdebski et al. Reference Izdebski, Słoczyński, Bonnier, Koloch and Kouli2020). The regional approaches provide new possibilities for providing models of full data series that can be helpful when looking at the potential impact of climate change on land-use strategies and anthropogenically induced land cover change.
Proxy records from speleothems
Speleothems (primarily stalagmites) from Greek caves have provided different types of proxies (Fig. 7.3). This data has been published in the past decade and offered significant new evidence for past climate variability (Kapsia: Finné et al. Reference Finné, Bar-Matthews, Holmgren, Sundqvist, Liakopoulos and Zhang2014; Alepotrypa: Boyd Reference Boyd2015; Mavri Trypa cave on Schiza island: Finné et al. Reference Finné, Holmgren, Shen, Hu, Boyd and Stocker2017; Skala Marion on Thasos: Psomiadis et al. Reference Psomiadis, Dotsika, Albanakis, Ghaleb and Hillaire-Marcel2018; see also Weiberg et al. Reference Weiberg, Unkel, Kouli, Holmgren, Avramidis, Bonnier, Dibble, Finné, Izdebski, Katrantsiotis, Stocker, Andwinge, Baika, Boyd and Heymann2016). Speleothem records are particularly useful in a Greek context, since several areas of the mainland are formed by limestone bedrock and caves containing speleothem are abundant. There is thus a great potential for expanding the volume of palaeoclimatic data from this type of archive and increasing the spatial and chronological resolution of climate reconstructions in the future. Speleothems form when surface water loaded with chemically weathered limestone bedrock enters a cave void and calcite is deposited. Calcite stalagmites drip-by-drip over long periods of time can be analysed for the composition of oxygen isotopes (δ18O). This proxy has, in a Mediterranean context, been interpreted to reflect variability precipitation amounts and soil moisture (Bar-Matthews et al. Reference Bar-Matthews, Ayalon, Gilmour, Matthews and Hawkesworth2003; Finné et al. Reference Finné, Bar-Matthews, Holmgren, Sundqvist, Liakopoulos and Zhang2014; Reference Finné, Holmgren, Shen, Hu, Boyd and Stocker2017; Cheng et al. Reference Cheng, Sinha, Verheyden, Nader, Li, Zhang, Yin, Yi, Peng, Rao, Ning and Edwards2015; Psomiadis et al. Reference Psomiadis, Dotsika, Albanakis, Ghaleb and Hillaire-Marcel2018).

7.3. Scan of the Kapsia speleothem cut in half for analysis. © M. Finné.
The chronology of the proxy data is defined by uranium-series dating (U-Th dating) (Richards and Dorale Reference Richards and Dorale2003). Uranium decays into thorium at a known rate and, by measuring the ratio between the two, the age of the sample can be determined. Under favourable circumstances (high uranium and low detrital input), chronologies for speleothems can be precise in comparison with radiocarbon dating, with two sigma (2σ) uncertainties only of around two decades. Proxy analyses in speleothems can be conducted at a sub-millimetre resolution, which, depending on the growth rate of the sample, can provide sub-decadal information about climate change (for a discussion on U-Th dating and radiocarbon dating and temporal resolution, see Finné and Weiberg Reference Finné, Weiberg, Ekblom, Isendahl and Lindholm2018).
Climate variability in the first millennium BC
Looking specifically at palaeoclimatic trajectories in the first millennium BC, the three published cave records from the Peloponnese offer a good starting point as they provide sequences that together span most or parts of the first millennium BC. Both the Alepotrypa and Kapsia caves provide complete datasets for this time frame, while in the Mavri Trypa record, there is a growth hiatus between 2952–2067 BP (i.e. 1002–117 BC). The latter also provides only limited data for the Hellenistic to Early Roman period (Finné et al. Reference Finné, Bar-Matthews, Holmgren, Sundqvist, Liakopoulos and Zhang2014; Reference Finné, Holmgren, Shen, Hu, Boyd and Stocker2017; Weiberg et al. Reference Weiberg, Unkel, Kouli, Holmgren, Avramidis, Bonnier, Dibble, Finné, Izdebski, Katrantsiotis, Stocker, Andwinge, Baika, Boyd and Heymann2016). This is unfortunate since the Mavri Trypa record has a high temporal resolution (number of samples per year) as well as relatively low dating uncertainty, unlike other records that have high sampling resolutions but also more pronounced dating uncertainties, with Kapsia being the most problematic (Finné et al. Reference Finné, Bar-Matthews, Holmgren, Sundqvist, Liakopoulos and Zhang2014; Finné and Weiberg Reference Finné, Weiberg, Ekblom, Isendahl and Lindholm2018).
In terms of the oxygen isotopes (δ18O) series, the records nevertheless provide broader trajectories that are similar to each other even if there are some discrepancies between shorter time-slices (Fig. 7.4 ). Above-average dry conditions seem to have prevailed in most of the Early Iron Age and Geometric periods, even if wetter spells also occur within this time frame. In the Archaic period, the signal seems to be more mixed, highlighting shifts between wetter and drier conditions at different times in the Kapsia and Alepotrypa records. The Classical to Early Hellenistic period, down to about 200 or 150 BC, is instead characterized by a generally humid climate, even if the Kapsia record suggests a pronounced dry spell in the first half of the fifth century BC. In the Kapsia record, dry conditions again seem to be present from the late second century BC going into the second century AD, while the Alepotrypa record suggests a dry spell slightly later, in the middle of the first century AD. The Mavri Trypa record is in turn difficult to interpret for the Late Hellenistic period due to the earlier growth hiatus but seems to indicate dry conditions also in the first century AD, even if the regrowth of the speleothem may suggest an increase of water entering the cave.

7.4. The δ18O series from the Kapsia, Mavri Trypa, and Alepotrypa speleothem records in the first millennium BC and first millennium AD. The shaded area highlights the duration of relative archaeological periods in the first millennium BC: PG = Protogeometric; EG–MG: Early to Middle Geometric; LG = Late Geometric. Adapted after Bonnier and Finné Reference Bonnier and Finné2020: fig. 2.
The trends provided by the Peloponnesian speleothems are not, however, mirrored in the data from the Skala Marion cave on Thasos in northern Greece (see the trend included in Fig. 7.5 ). Here we can observe almost the opposite signal for the late second and first millennium BC: the period between 1450 and 750 BC is largely characterized by humid climate conditions without any indications of the arid conditions seen in the other records for the Late Bronze Age and the Early Iron Age. A shift towards a drier climate can instead be observed in the second half of the first millennium BC, after which the oxygen isotopes (δ18O) indicate humid conditions moving into the first centuries AD (Psomiadis et al. Reference Psomiadis, Dotsika, Albanakis, Ghaleb and Hillaire-Marcel2018). The differences between the climate signal from the Peloponnesian cave archives and the Skala Marion speleothem are interesting and can be attributed either to regional differences in climate or ambiguities in proxy interpretation or to dating inaccuracies. These differences show the importance of using local records as part of archaeological research. It is, however, interesting that a recently published record from the Kocain cave in southwestern Turkey shows close similarities to the Peloponnesian records. The trends from Kocain in terms of precipitation through measurements of oxygen isotopes (δ18O) and effective moisture utilizing magnesium to calcium ratios (Mg/Ca) are, in particular, close to the trends seen in the isotope data from Kapsia (Jacobson et al. Reference Jacobson, Flohr, Gascoigne, Leng, Sadekov, Cheng, Edwards, Tüysüz and Fleitmann2021).

7.5. A comparison of proxy series from lake and speleothem archives: (a) geochemical proxies/PC1, Stymphalos (Peloponnese); (b) geochemical proxies/PC1, Kaisari (Peloponnese); (c) geochemical proxies/PC1, Pheneos (Peloponnese); (d) geochemical proxies/PC1, Asea (Peloponnese); (e) geochemical proxies/PC1, Gialova (Peloponnese); (f) δD23, Lerna, Peloponnese (Greece), (g) δD23, Agios Floros, Peloponnese; (h) δ18O, Mavri Trypa, Schiza Island; (i) δ18O, Skala Marion, Thasos; (j) autumn–winter precipitation, Closani cave (Romania), (k) Balkan synthesis based on z-scores based on 10 sedimentary archives. Shaded red areas indicate periods of potentially drier or warmer climate (RWP = Roman warm period; MWP = Medieval warm period). Seguin et al. Reference Seguin, Avramidis, Haug, Kessler, Schimmelmann and Unkel2020b: fig. 8.
The climate signals provided by the lake and wetland archives offer a much more heterogenous picture, even within the Peloponnese. The recent comparison of multiple lake records based on PC1 ratios carried out by Joana Seguin and colleagues is telling (Seguin et al. Reference Seguin, Avramidis, Haug, Kessler, Schimmelmann and Unkel2020b) (Fig. 2.5). The humid conditions visible in the Classical and Hellenistic period down to the second century BC, as suggested by the cave records, are not always reflected in the lake records, where we seem to observe instead increasing aridity and/or higher temperatures already in the fourth century BC at both Pheneos and Asea, while the climate signal seems to be more humid and stable at both Kaisari and Stymphalos at this time (Seguin et al. Reference Seguin, Avramidis, Haug, Kessler, Schimmelmann and Unkel2020b; but for Asea, see also Unkel et al. Reference Unkel, Schimmelmann, Shriner, Forsén, Heymann and Brückner2014). At Lake Lerna, the analyses of stable isotopes from n-alkanes also point towards increasingly dry conditions in the Classical and Hellenistic periods, culminating in a very dry spell between the fourth and first century BC (Katrantsiotis et al. Reference Katrantsiotis, Norström, Smittenberg, Finné, Weiberg, Hättestrand, Avramidis and Wastegård2019).
A dry spell in the second half of the first millennium BC is further suggested by biological proxies (microfauna) analysed from Lake Keri, Zakynthos (Avramidis et al. Reference Avramidis, Kalaitzidis, Iliopoulos, Papadopoulou, Nikolaou, Papazisimou, Christanis and Van Wijngaarden2017). However, in the southwestern Peloponnese, some slightly different trajectories can be inferred from multiproxy analyses of lake sediment cores. At Aghios Floros, the analysis of diatoms and geochemical elements indicates that accumulation rates seem to increase in around 850 BC following a period of dry conditions. Also, the appearance of benthic and planktonic species in the middle of the first millennium BC is indicative of the formation of a shallow body of water in the marshland, which, in turn, points to increasingly humid conditions (Katrantsiotis et al. Reference Katrantsiotis, Norström, Holmgren, Risberg and Skelton2016). The analysis of stable isotopes from n-alkanes of both terrestrial and aquatic plants at Aghios Floros also suggests dry conditions in the initial part of the first millennium BC followed by increasingly humid conditions in the Late Geometric period and onwards (Norström et al. Reference Norström, Katrantsiotis, Smittenberg and Kouli2017; Reference Norström, Katrantsiotis, Finné, Risberg, Smittenberg and Bjursäter2018).
Further west in Messenia, the recorded Rb/Sr ratio in the Gialova lagoon suggests that dry conditions were present from the Late Bronze Age down to around 500 BC (Emmanouilidis et al. Reference Emmanouilidis, Katrantsiotis, Norström, Risberg, Kylander, Sheik, Iliopoulos and Avramidis2018), but the study of isotopes from n-alkanes of terrestrial plans (δD31) nuances these results and instead suggests a trend towards wetter and/or colder conditions in the period between ca 1050–750 BC. In the Archaic and Classical periods, down to the middle of the fourth century BC, the record suggests increasingly dry and/or warm conditions, but these conditions do not reach the arid conditions of the Late Bronze Age (Katrantsiotis et al. Reference Katrantsiotis, Kylander, Smittenberg, Yamoah, Hättestrand, Avramidis, Strandberg and Norström2018).
North of the Peloponnese, at the Aliki salt march (by ancient Siphai on the western coast of Boiotia), for example, a multiproxy analysis indicates humid conditions in the second half of the first millennium BC, but human activities in the catchment of the marshland have also had a clear impact on the record, suggesting that any palaeoclimatic interpretation should be made with caution (Emmanouilidis et al. Reference Emmanouilidis, Unkel, Triantaphyllou and Avramidis2020b). The PC1 and Rb/Sr ratio from a core extracted at Lake Trichonida in Aitolia highlight changes in the hydrological environment indicating wet conditions in the Archaic period. After the Archaic period the record suggests instead slight shifts between drier and more humid conditions in the Classical period, followed by a progression towards drier conditions that reach their peak in the Early Roman period (Seguin et al. Reference Seguin, Avramidis, Dörfler, Emmanouilidis and Unkel2020a).
The arid conditions in the Late Hellenistic and Early Roman periods as suggested by the cave records are to a certain extent also highlighted by the data from the lake records, even if dry conditions seem to commence earlier in some of the archives in the northern and central Peloponnese. Dry conditions in the first centuries AD, are, for example, suggested by both hydrogen isotopes (δ18Obulk) and geochemical proxies from Lake Vouliagmeni on the Perachora Peninsula in the northern Peloponnese, whereas the same record provides evidence of wetter conditions in the Classical period (Emmanouilidis et al. Reference Emmanouilidis, Katrantsiotis, Dotsika, Kokkalas, Unkel and Avramidis2022a). At both Gialova and Aghios Floros in Messenia, there is also a shift towards drier conditions in the first centuries AD based on the multiproxy analysis (Norström et al. Reference Norström, Katrantsiotis, Smittenberg and Kouli2017; Reference Norström, Katrantsiotis, Finné, Risberg, Smittenberg and Bjursäter2018; Katrantsiotis et al. Reference Katrantsiotis, Kylander, Smittenberg, Yamoah, Hättestrand, Avramidis, Strandberg and Norström2018). However, at the Kaisari polje in the northern Peloponnese, the signal indicates a continuation of stable and slightly wetter conditions in the Early Roman period (Seguin et al. Reference Seguin, Avramidis, Haug, Kessler, Schimmelmann and Unkel2020b). Similarly, at Asea the geochemical proxies suggest a shift towards wetter and/or colder climate conditions in the first centuries AD (Unkel et al. Reference Unkel, Schimmelmann, Shriner, Forsén, Heymann and Brückner2014).
The diverse signals provided by different proxy records from the Peloponnese emphasize that it is difficult to build a general model of climate and climate change in the first millennium BC. The differences between the signals could reflect actual differences between local climate trajectories, but the impact of anthropogenic land use in the lake catchments must also be considered as a potential factor, particularly concerning the geochemical proxies, as discussed above. The differences between records could also be at least partly linked to the chronological uncertainties and differences in terms of chronological resolution. Geochemical proxies and the PC1 trends are provided at a higher temporal resolution compared to stable isotope data of n-alkanes (as discussed by Seguin et al. Reference Seguin, Avramidis, Dörfler, Emmanouilidis and Unkel2020a). The differences between archives and regional variations can also be tied to atmospheric circulation patterns that impact regional variations in precipitation and temperature in the Greek mainland between the drier east and the more humid west (Katrantsiotis et al. Reference Katrantsiotis, Norström, Smittenberg, Finné, Weiberg, Hättestrand, Avramidis and Wastegård2019; Seguin et al. Reference Seguin, Avramidis, Dörfler, Emmanouilidis and Unkel2020a; Post Reference Post2022b).
When reading scientific reports on the proxy data, it is, however, not always straightforward to understand what the signals convey in terms of either temperature or hydroclimate. Most records nevertheless seem to be linked to precipitation variability, which is important in terms of ancient agriculture. This was dependent on winter and spring precipitation for cultivation. Grains (both wheat and barley) have thresholds connected to annual rainfall that will affect the output of the crops (Aschonitis et al. Reference Aschonitis, Lithourgidis, Damalas and Antonopoulos2013; Bonnier and Finné Reference Bonnier, Finné, Erdkamp, Manning and Verboven2021, with references). In years with drier conditions the output may be severely impacted, especially in the naturally drier areas of the Greek mainland, such as Attica and the northeastern Peloponnese. Olives are difficult to place in a specific climatological context, as they are, to a large extent, resilient in dry climates and more sensitive to cold temperatures and winter frosts but can nevertheless be affected by changes in precipitation. The output of olives is generally higher from trees situated in areas that receive between 400mm and 600mm of rainfall annually, even if cultivation can be successful in areas with lower rainfall. Severe droughts in ‘off years’ can, however, impact the biennial cycle and hinder the growth of wood, which can also impact yields in coming years (Foxhall Reference Foxhall2007).
The expansion in the more humid phases of the first millennium BC of agricultural land use and the increased use of sloping ground with thinner soil cover can thus be suitably matched with an increase in olive cultivation (Bonnier and Finné Reference Bonnier and Finné2020; Reference Bonnier, Finné, Erdkamp, Manning and Verboven2021). Both individual archives and syntheses of pollen records suggest an expansion of olive cultivation in the seemingly humid Archaic, Classical, and Early Hellenistic periods followed by a subsequent reduction in the proportion of olea in the possibly drier Late Hellenistic and Roman period ( Brauron: Kouli Reference Kouli2012; Eleusis Bay (marine core SP2): Kyrikou et al. Reference Kyrikou, Kouli, Triantaphyllou, Dimiza, Gogou, Panagiotopoulos, Anagnostou and Karageorgis2020; multiple archives: Weiberg et al. Reference Weiberg, Bevan, Kouli, Katsianis, Woodbridge, Bonnier, Engel, Finné, Fyfe, Maniatis, Palmisano, Panajiotidis, Roberts and Shennan2019; Izdebski et al. Reference Izdebski, Słoczyński, Bonnier, Koloch and Kouli2020). These trends are also synchronic with the contraction of land use away from areas with thinner soil cover (Bonnier and Finné Reference Bonnier, Finné, Erdkamp, Manning and Verboven2021), but care should be taken not to overemphasize these general trends and, as always, local records are important to nuance the picture.
The recent palynological analysis of a new core extracted at Lake Lerna shows an expansion in the proportion of olive cultivation during the Roman period (Vignola et al. Reference Vignola, Hättestrand, Bonnier, Finné, Izdebski, Katrantsiotis, Kouli, Liakopoulos, Norström, Papadaki, Strandberg, Weiberg and Masi2022). Similarly, at Lake Kournas on Crete, there is no substantial change in land use during the Roman period and olive cultivation is maintained in high proportions throughout this period (Jouffroy-Bapicot et al. Reference Jouffroy-Bapicot, Pedrotta, Debret, Field, Sulpizio, Zanchetta, Sabatier, Roberts, Tinner, Walsh and Vannière2021). As in the case of the climate proxies, it is important to consider the local context of the land-use trajectories suggested by the pollen data. The geomorphology and topographic environment of the lake catchments could, for example, influence the possible impact that a shift in climate may have had on cultivation patterns. An increase in the volume of available high-resolution palaeoenvironmental records can therefore help to distinguish between local and regional trajectories and, in combination with archaeology, can provide a more multifaceted picture of climate and socio-environmental dynamics in the Greek world.
Conclusion: moving forward
The past decade has seen a substantial increase in the volume of research surrounding human–environment interactions in the Greek world as well as a rapid growth in the volume of available proxy data, particularly from the Peloponnese. Recent research highlights above all the synchronicity of different environmental and socioeconomic processes, as seen from both palaeoenvironmental proxies and the archaeological record. In particular, long-term perspectives have been useful in identifying patterns and recurrent issues linked to both dry and wet climate phases. The chronological uncertainties, inherent in all records, nevertheless emphasize the need for an increase in the volume of reliable proxy data with both high temporal resolution and lower chronological uncertainties to provide more secure comparisons of archaeological and palaeoenvironmental datasets through chronology. New records from sediment cores and speleothems from multiple areas of Greece and the Aegean would thus help to provide a broader picture of regional climate trajectories as well as show local variations within these trajectories. Any improvements in the chronology of archaeological datasets through new C14 dates and refined ceramic chronologies would be equally useful to enhance data comparison, as the archaeological data often sets the limits of chronological resolution of the integrated analyses.
But comparative research based solely on the comparison of times series is not enough. We also need to think further about how climate change can impact socioeconomic processes and stimulate societal responses at different societal scales. Such dynamics need to be considered at multiple scales – from the household level to regional settlement structures and associated land use – and we need to develop further methods for such integrated research. Here archaeology must play a key role. In particular, we need to improve the archaeological record and explore questions on climate and human–environment interactions when designing archaeological projects and archaeological sampling strategies. We need to increase the volume of archaeobotanical and zooarchaeological evidence arising from excavated contexts of different site types (both urban and rural) from all periods. This will help us to examine how consumption patterns and agricultural strategies may, or may not, have been impacted by climate variability. New scientific analyses of faunal and plant remains are also crucial. Stable isotope analyses can, for example, provide diachronic evidence for agricultural production strategies, patterns of manuring and irrigation as well as the seasonal mobility of herds, which is highly important for future discussions on past climate change and societal responses.