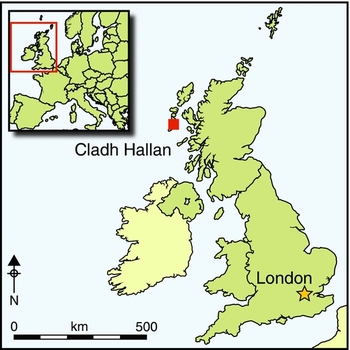
Introduction
In previous papers in Antiquity, we have presented the first evidence for mummification in prehistoric Britain (Parker Pearson et al. Reference Parker Pearson, Chamberlain, Craig, Marshall, Mulville, Smith, Chenery, Collins, Cook, Craig, Evans, Hiller, Montgomery, Schwenninger, Taylor and Wess2005, Reference Parker Pearson, Chamberlain, Collins, Cox, Craig, Craig, Hiller, Marshall, Mulville and Smith2007). Mummification is defined here as the preservation of bodily soft tissue via natural processes (e.g. deposition of a corpse within a preservative environment such as a sphagnum peat bog) or artificial means (e.g. embalming) (Aufderheide Reference Aufderheide2003: 41). Skeletons recovered from beneath Late Bronze Age roundhouses at Cladh Hallan on South Uist, Western Isles of Scotland, were shown to have been mummified prior to deposition (Parker Pearson et al. Reference Parker Pearson, Chamberlain, Craig, Marshall, Mulville, Smith, Chenery, Collins, Cook, Craig, Evans, Hiller, Montgomery, Schwenninger, Taylor and Wess2005, Reference Parker Pearson, Chamberlain, Collins, Cox, Craig, Craig, Hiller, Marshall, Mulville and Smith2007, Reference Parker Pearson, Cox Willis, Marshall, Mulville, Smith, Cowie, Craig, Deluis, Juddery, Manley, Schwenninger and Taylor2013); the osteological and ancient DNA analyses also indicate that these ostensibly articulated single individuals had been reconstructed from the preserved anatomical parts of several people (Parker Pearson et al. Reference Parker Pearson, Chamberlain, Craig, Marshall, Mulville, Smith, Chenery, Collins, Cook, Craig, Evans, Hiller, Montgomery, Schwenninger, Taylor and Wess2005; Hanna et al. Reference Hanna, Bouwman, Brown, Parker Pearson and Brown2012). These findings raise questions about the extent, distribution and nature of mummification in prehistoric Britain, a difficult research area given that similar circumstances of preservation and recovery to those found at Cladh Hallan are unlikely to be present in most parts of Britain or Europe. Our aim has been to develop a single method of analysis that can be used consistently to identify previously mummified skeletons more widely.
Microscopic analysis of bone histology was one of the main methods used to infer mummification at Cladh Hallan. The most common, almost ubiquitous, form of diagenetic alteration observed within archaeological bone microstructure consists of bioerosive tunnelling produced by invasive microorganisms (Hackett Reference Hackett1981; Hedges Reference Hedges2002; Turner-Walker et al. Reference Turner-Walker, Nielsen-Marsh, Syversen, Kars and Collins2002; Jans et al. Reference Jans, Nielsen-Marsh, Smith, Collins and Kars2004; Nielsen-Marsh et al. Reference Nielsen-Marsh, Smith, Jans, Nord, Kars and Collins2007; Figure 1). There is a growing body of evidence indicating that this bacterial bioerosion is produced by an organism's intrinsic gut bacteria during putrefaction (Child Reference Child1995; Bell et al. Reference Bell, Skinner and Jones1996; Jans et al. Reference Jans, Nielsen-Marsh, Smith, Collins and Kars2004; Guarino et al. Reference Guarino, Angelini, Vollono and Orefice2006; Nielsen-Marsh et al. Reference Nielsen-Marsh, Smith, Jans, Nord, Kars and Collins2007; White & Booth Reference White and Booth2014), suggesting that bacterial bioerosion of archaeological bone reflects the extent of bodily putrefaction experienced during the early post-mortem stages.
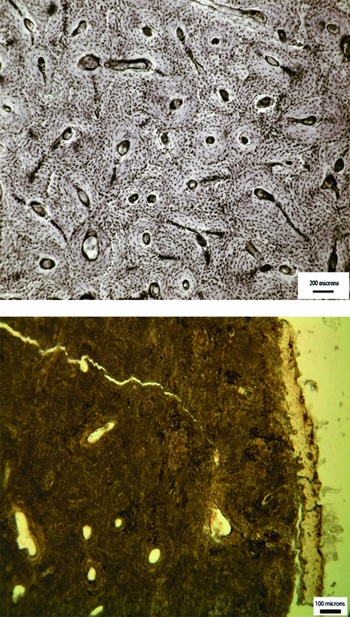
Figure 1. Transmitted light micrograph of a human, fresh bone transverse femoral thin section (top), demonstrating perfect microstructural preservation and a typical archaeological femoral section (bottom) where the internal microstructure has been extensively altered by bacteria.
Histological analysis of the femur of Cladh Hallan skeleton 2638, a composite adult male (Parker Pearson et al. Reference Parker Pearson, Chamberlain, Craig, Marshall, Mulville, Smith, Chenery, Collins, Cook, Craig, Evans, Hiller, Montgomery, Schwenninger, Taylor and Wess2005), revealed that it had been subjected to only limited levels of bacterial bioerosion, indicating that initial putrefactive activity was arrested. A similar conclusion was reached for the composite female-male skeleton 2613 (Parker Pearson et al. Reference Parker Pearson, Chamberlain, Craig, Marshall, Mulville, Smith, Chenery, Collins, Cook, Craig, Evans, Hiller, Montgomery, Schwenninger, Taylor and Wess2005, Reference Parker Pearson, Cox Willis, Marshall, Mulville, Smith, Cowie, Craig, Deluis, Juddery, Manley, Schwenninger and Taylor2013). The condition of these two composite human skeletons contrasts with results of previous microscopic studies on archaeological articulated human bones, which usually show extensive tunnelling by bacteria (Hedges Reference Hedges2002; Jans et al. Reference Jans, Nielsen-Marsh, Smith, Collins and Kars2004; Nielsen-Marsh et al. Reference Nielsen-Marsh, Smith, Jans, Nord, Kars and Collins2007). By contrast, faunal bones recovered from the same machair (shell sand) sediments at Cladh Hallan demonstrated extensive bacterial alteration (Parker Pearson et al. Reference Parker Pearson, Chamberlain, Craig, Marshall, Mulville, Smith, Chenery, Collins, Cook, Craig, Evans, Hiller, Montgomery, Schwenninger, Taylor and Wess2005; Mulville et al. Reference Mulville, Madgwick, Powell, Parker Pearson and Pluskowski2011).
Putrefaction is a highly destructive process and the most successful methods of mummification neutralise or remove visceral bacteria to prevent this stage of bodily decomposition (Aufderheide Reference Aufderheide2003). Putrefactive bacteria are likely to include osteolytic species responsible for bioerosion (Bell et al. Reference Bell, Skinner and Jones1996; Jans et al. Reference Jans, Nielsen-Marsh, Smith, Collins and Kars2004), therefore bacterial attack can be expected to be absent or limited within bones from mummified bodies. The arrested pattern of bacterial bioerosion observed within the Cladh Hallan skeleton is theoretically consistent with mummification. Consequently, microscopic investigation may be the best and most consistent method for identifying previously mummified skeletons.
A diagenetic signature for mummification?
In most cases, previous investigations of the bone histology of bona fide mummified archaeological remains (Table 1) have not reported directly or in detail on histological preservation. The descriptions of samples and their images reveal, however, that mummified bones usually demonstrate immaculate levels of histological preservation. These results support the hypothesis that ancient mummified bones are unlikely to have been affected by putrefactive bioerosion. This typical absence of bacterial bioerosion in known mummified bone is not entirely consistent with the arrested pattern of attack observed within the Cladh Hallan skeletons.
Table 1. Catalogue of ancient human mummies whose bones have been subject to histomorphological analysis.

Those mummified bodies examined in previous histomorphological studies are preserved in ways that would have affected putrefaction immediately after death (Weinstein et al. Reference Weinstein, Simmons and Lovejoy1981; Thompson & Cowen Reference Thompson and Cowen1984; Stout Reference Stout1986; Brothwell & Bourke Reference Brothwell, Bourke, Turner and Scaife1995; Garland Reference Garland, Turner and Scaife1995; Hess et al. Reference Hess, Klima, Pfaller, Künzel and Gaber1998; Monsalve et al. Reference Monsalve, Humphrey, Walker, Cheung, Vogl and Nimmo2008; Bianucci et al. Reference Bianucci, Brothwell, van der Sanden, Papgeorgopoulou, Gostner, Pernter, Egarter-Vigl, Maixner, Janko, Piombino-Mascali, Mattutino, Rühli and Zink2012). The evidence for the onset and subsequent halting of putrefaction in the Cladh Hallan bodies suggests that the method of mummification employed here had an inconsistent or delayed effect on bodily decomposition (Parker Pearson et al. Reference Parker Pearson, Chamberlain, Craig, Marshall, Mulville, Smith, Chenery, Collins, Cook, Craig, Evans, Hiller, Montgomery, Schwenninger, Taylor and Wess2005). To test the relationship between bone bioerosion and the extent of soft tissue preservation, the microstructures of bone samples from a mummy and a bog body were examined using thin-section light microscopy. These samples consist of the patella of a desiccated prehistoric mummy, retrieved from the town of Kawkaban in northern Yemen, and the tibia of a partially mummified Bronze Age body recovered from a sphagnum peat bog at Derrycashel in County Roscommon, Ireland. We made applications to analyse bone from a range of mummified remains; we were, however, granted access to sample only these two specimens.
The soft tissue preservation of the Yemeni individual suggests that putrefaction was arrested soon after death because of the arid environment (Don Brothwell pers. comm.). Bodies placed in arid environments dry out rapidly, depriving putrefactive bacteria of essential moisture (Aufderheide Reference Aufderheide2003). Only the top half of the Derrycashel bog body retained soft tissue and it is likely that it had putrefied to some extent before decomposition was curtailed by the preservative chemicals in the bog environment (Eamonn Kelly pers. comm.).
Thin sections of the mummified bones were assessed using the standard Oxford Histological Index (OHI), which translates the percentage of remaining intact bone microstructure into an ordinal scale ranging from 0 (worst preserved) to 5 (best preserved) (Hedges et al. Reference Hedges, Millard and Pike1995; Millard Reference Millard, Brothwell and Pollard2001). The histological preservation of the Yemeni mummified patella is excellent (OHI = 5), although enlarged osteocyte lacunae (natural cavities in the bone microstructure that house osteocyte cells) were observed towards the periosteal (outer) surface (Figure 2). Post-mortem enlargement of osteocyte lacunae has been linked to acidic erosion, staining by exogenous substances and the initial stages of bacterial bioerosion (Gordon & Buikstra Reference Gordon and Buikstra1981; Garland Reference Garland, Boddington, Garland and Janaway1987; Bell et al. Reference Bell, Skinner and Jones1996; Turner-Walker & Peacock Reference Turner-Walker and Peacock2008; White & Booth Reference White and Booth2014).
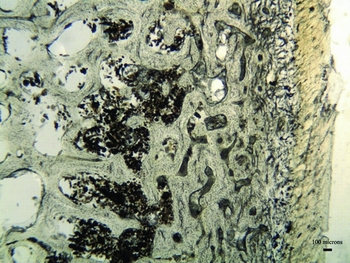
Figure 2. Micrograph of the patella thin section from a Yemeni mummy demonstrating immaculate histological preservation (soft tissue can be observed adhering to the periosteal surface to the right of the image).
It is unlikely that the attack observed within the Yemeni patella was a result of acidic erosion because the bone was still protected by soft tissue; there were no other typical signs of acidic degradation such as microfissuring, while the distribution of attack did not form a characteristic diffuse wave of destruction (Gordon & Buikstra Reference Gordon and Buikstra1981; Turner-Walker & Peacock Reference Turner-Walker and Peacock2008). Acidic degradation would normally result in the destruction of the whole bone over an archaeological timescale (Nielsen-Marsh et al. Reference Nielsen-Marsh, Smith, Jans, Nord, Kars and Collins2007; Smith et al. Reference Smith, Nielsen-Marsh, Jans and Collins2007). Enlarged osteocyte lacunae caused by exogenous staining are usually accompanied by discolouration of the surrounding bone microstructure (Garland Reference Garland, Boddington, Garland and Janaway1987; Schultz Reference Schultz, Haglund and Sorg1997). Such discolouration was not apparent within the Yemeni sample. Therefore, the best explanation for the enlarged osteocyte lacunae observed within the Yemeni patella is that it was exposed to initial putrefactive activity, which was rapidly curtailed (Bell et al. Reference Bell, Skinner and Jones1996; Jans et al. Reference Jans, Nielsen-Marsh, Smith, Collins and Kars2004; Hollund et al. Reference Hollund, Jans, Collins, Kars, Joosten and Kars2012).
Histologically, the thin section of the Derrycashel tibia shows the bone to be well-preserved (OHI = 5), but it displays numerous enlarged osteocyte lacunae within the sub-periosteal zone that have amalgamated to form larger areas of alteration consistent with bacterial bioerosion (Hackett Reference Hackett1981; Figure 3). The survival of the whole bone and the distribution of attack are inconsistent with acidic erosion or staining. The Derrycashel sample demonstrates lower levels of bacterial bioerosion than were observed in the Cladh Hallan specimen, but, overall, this result suggests that the Cladh Hallan diagenetic signature is indeed consistent with mummification using a technique that promoted partial soft-tissue preservation. The Cladh Hallan skeletons were recovered from alkaline machair sediments, yet previous Fourier transform infrared (FTIR) and small-angle X-ray scattering (SAXS) analyses of these specimens had indicated that bone mineral crystals located towards the periosteal (external) surfaces had been altered in a manner consistent with acidic chemical dissolution (Parker Pearson et al. Reference Parker Pearson, Chamberlain, Craig, Marshall, Mulville, Smith, Chenery, Collins, Cook, Craig, Evans, Hiller, Montgomery, Schwenninger, Taylor and Wess2005). This result was used to suggest that mummification may have been achieved through deposition within an acidic peat bog (Parker Pearson et al. Reference Parker Pearson, Chamberlain, Craig, Marshall, Mulville, Smith, Chenery, Collins, Cook, Craig, Evans, Hiller, Montgomery, Schwenninger, Taylor and Wess2005); the evidence from the Derrycashel bog body provides further support for this hypothesis. The histological analysis of the Kawkaban and Derrycashel samples is in agreement with previous microscopic studies of mummified bone and suggests that mummification prevents or limits putrefactive bioerosion of the skeleton, producing a characteristic diagenetic signature.

Figure 3. Micrograph of the tibial thin section from the Derrycashel individual; the tannins within the bog environment have stained the bone red; the microstructure is well-preserved, but limited accumulations of bacterial tunnelling (black areas) can be observed towards the periosteal surface.
Identification of further Bronze Age mummies
The use of measurements of bacterial bioerosion to interpret post-mortem treatment of a body is hampered by problems of equifinality. Bones from anoxic or waterlogged environments often display patterns of arrested bacterial bioerosion similar to those from mummified remains (Janaway Reference Janaway, Hunter, Roberts and Martin1996; Turner & Wiltshire Reference Turner and Wiltshire1999; Turner-Walker & Jans Reference Turner-Walker and Jans2008; Hollund et al. Reference Hollund, Jans, Collins, Kars, Joosten and Kars2012); microscopic analysis cannot therefore be used to infer previous mummification within skeletons recovered from these contexts. Neonatal bones may naturally remain free from bacterial bioerosion after death, as the mammalian gut microbiome only develops in the days after birth (Jans et al. Reference Jans, Nielsen-Marsh, Smith, Collins and Kars2004; White & Booth Reference White and Booth2014). Excarnation promotes rapid exogenous skeletonisation and disarticulation by carnivorous insects and limits the impact of soft-tissue putrefaction on the skeleton (Rodriguez & Bass Reference Rodriguez and Bass1983; Bell et al. Reference Bell, Skinner and Jones1996; Fernández-Jalvo et al. Reference Fernández-Jalvo, Andrews, Pesquero, Smith, Marín-Monfort, Sánchez, Geigl and Alonso2010; Simmons et al. Reference Simmons, Cross, Adlam and Moffatt2010; White & Booth Reference White and Booth2014). Dismemberment, defleshing and other processes that separate the bone from the gut bacteria would also produce disarticulated bones that display limited degrees of bacterial attack (Jans et al. Reference Jans, Nielsen-Marsh, Smith, Collins and Kars2004; Nielsen-Marsh et al. Reference Nielsen-Marsh, Smith, Jans, Nord, Kars and Collins2007). Given the rapidity of skeletal disarticulation that accompanies bodily decomposition, the most obvious way in which an articulated skeleton can survive archaeologically is through immediate burial of the corpse (Duday Reference Duday, Gowland and Knüsel2006). Burial protects the body from skeletonising insects, and bones from buried bodies typically exhibit advanced bioerosion resulting from extensive putrefaction of soft tissues, in contrast to reduced or absent bioerosion resulting from mummification (Rodriguez & Bass Reference Rodriguez and Bass1985; Rodriguez Reference Rodriguez, Haglund and Sorg1997).
A microscopic study of archaeological human long-bone thin sections (97% femora) representing 301 individuals retrieved from 25 European sites, of which 24 were British, found that bacterial bioerosion relates to funerary treatment in predictable ways based on models of bodily decomposition (Booth Reference Booth2014). Most samples of bone retrieved from historic-period contexts (Roman and later), where there is good evidence that these individuals were buried soon after death, produced the lowest OHI score of 0 (typified in Figure 1) and almost all scored less than 2. Less than 3% demonstrated the high OHI scores of 4 or 5 assigned to the Cladh Hallan skeletons and the mummified specimens. These findings suggest that skeletons of mummified bodies are the only ancient articulated remains that either consistently remain free from bacterial bioerosion or demonstrate only limited levels of bacterial attack. Microscopic examination of bioerosion in articulated skeletons thus provides a plausible method for identifying past mummification.
The patterns of bacterial bioerosion observed amongst Bronze Age skeletons, with the addition of a further 6 individuals from Canada Farm, Dorset, were remarkably distinctive compared with the results from the historical, Neolithic and Iron Age assemblages (Figure 4a). Just over half of the Bronze Age samples (18 out of 34) produced low OHI scores consistent with immediate burial, but the remainder produced high scores of 4 or 5, indicating excellent bone preservation comparable with the mummified examples. Most of these high-scoring samples are free from bacterial bioerosion. A significantly large proportion of Bronze Age bones had been subject to early post-mortem processes that had limited their exposure to putrefaction bacteria. Two of these were recovered from waterlogged sediments at Bradley Fen in Cambridgeshire (Gibson & Knight Reference Gibson and Knight2006) and Langwell Cist in Strath Oykel (Lelong Reference Lelong2009, Reference Lelong2012). Additionally, some of the other high-scoring Bronze Age human remains were recovered in various stages of skeletal disarticulation (Bell et al. Reference Bell, Skinner and Jones1996; Fernández-Jalvo et al. Reference Fernández-Jalvo, Andrews, Pesquero, Smith, Marín-Monfort, Sánchez, Geigl and Alonso2010; Simmons et al. Reference Simmons, Cross, Adlam and Moffatt2010).
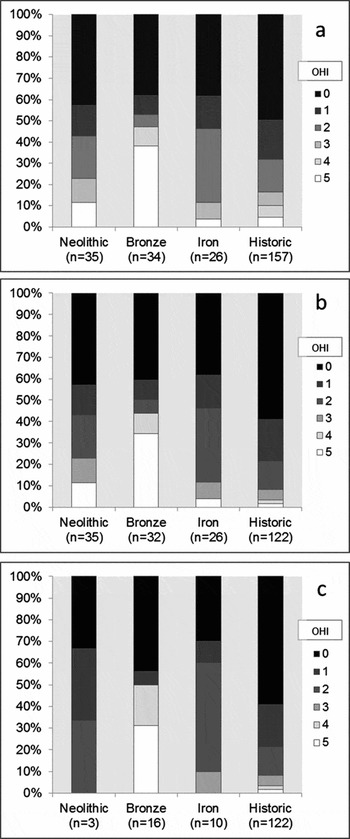
Figure 4. Distribution of Oxford Histological Index (OHI) scores amongst: a) post-neonatal bones; b) post-neonatal bones from aerobic environments; c) articulated post-neonatal bones from aerobic environments, separated by phase; significant proportions of Bronze Age samples retain high OHI scores in each case.
The exclusion of waterlogged and disarticulated bone samples does not, however, affect the overall distinctive distribution of Bronze Age OHI scores (Figure 4b & c). The Bronze Age sample set from aerobic environments is distributed evenly between articulated (n = 16) and disarticulated (n = 16) skeletons. The regular occurrence of histologically well-preserved articulated human bone samples is exclusive to the Bronze Age sample. Only 3 of the 35 Neolithic samples originate from articulated skeletons. All articulated Neolithic bone samples are extensively bioeroded, but the possibility that a proportion of Neolithic articulated skeletons will demonstrate high levels of histological preservation cannot be dismissed entirely. The distribution of variably articulated skeletons amongst the Iron Age sample set was more balanced (10 articulated, 16 disarticulated).
Instances of well-preserved Bronze Age bone were identified from remains at several different sites, removing the possibility that these results are attributable to the disproportionate influence of one large but anomalous sample set. It is highly unlikely that sampling of a small number of Bronze Age individuals from a varied group of sites would have repeatedly captured anomalous specimens. Most Bronze Age sites that have yielded histologically well-preserved bones also provide examples of extensively bioeroded remains. In all cases, these contrasting samples originate from skeletons found only a few metres apart, within similar sediments. These results suggest that histological bone preservation has not been dictated by either specific environmental conditions or exogenous soil bacteria (Fernández-Jalvo et al. Reference Fernández-Jalvo, Andrews, Pesquero, Smith, Marín-Monfort, Sánchez, Geigl and Alonso2010; Turner-Walker Reference Turner-Walker2012). The unconventional arrested patterns of bioerosion observed amongst samples of articulated Bronze Age skeletons must relate to an early anthropogenic process that limited bodily putrefaction (Bell et al. Reference Bell, Skinner and Jones1996 ; Jans et al. Reference Jans, Nielsen-Marsh, Smith, Collins and Kars2004; Nielsen-Marsh et al. Reference Nielsen-Marsh, Smith, Jans, Nord, Kars and Collins2007). Mummification represents the only plausible method of significantly reducing the deleterious effects of bodily putrefaction while retaining skeletal articulation. Mummified bodies are the only articulated archaeological remains to demonstrate consistently the diagenetic pattern observed amongst the Bronze Age samples, the simplest explanation is, therefore, that a substantial proportion of these bodies were mummified before they were buried.
All of the histologically well-preserved disarticulated Bronze Age bones were free from bacterial bioerosion. Sub-aerial exposure could be responsible for this result, although bones from exposed carcasses usually demonstrate some bacterial bioerosion; skeletonisation in temperate environments is rarely quick enough to prevent the bones from experiencing soft-tissue putrefaction altogether (Bell et al. Reference Bell, Skinner and Jones1996; Fernández-Jalvo et al. Reference Fernández-Jalvo, Andrews, Pesquero, Smith, Marín-Monfort, Sánchez, Geigl and Alonso2010; Simmons et al. Reference Simmons, Cross, Adlam and Moffatt2010; Hollund et al. Reference Hollund, Jans, Collins, Kars, Joosten and Kars2012; White & Booth Reference White and Booth2014). Immaculate histological bone preservation is more consistent with mummification than with excarnation (Weinstein et al. Reference Weinstein, Simmons and Lovejoy1981; Thompson & Cowen Reference Thompson and Cowen1984; Stout Reference Stout1986; Brothwell & Bourke Reference Brothwell, Bourke, Turner and Scaife1995; Hess et al. Reference Hess, Klima, Pfaller, Künzel and Gaber1998). When it is considered that the Cladh Hallan bodies were constructed out of the partially disarticulated elements of several individuals (Parker Pearson et al. Reference Parker Pearson, Chamberlain, Craig, Marshall, Mulville, Smith, Chenery, Collins, Cook, Craig, Evans, Hiller, Montgomery, Schwenninger, Taylor and Wess2005; Reference Parker Pearson, Chamberlain, Collins, Cox, Craig, Craig, Hiller, Marshall, Mulville and Smith2007; Reference Parker Pearson, Cox Willis, Marshall, Mulville, Smith, Cowie, Craig, Deluis, Juddery, Manley, Schwenninger and Taylor2013; Hanna et al. Reference Hanna, Bouwman, Brown, Parker Pearson and Brown2012), the most parsimonious interpretation of all of the histologically well-preserved Bronze Age bone samples is that they represent parts of, or whole, previously mummified individuals.
Archaeological bones from intermittently waterlogged environments demonstrate variably elevated levels of histological preservation, most likely corresponding with the varying degree of bodily decomposition that took place before the grave was inundated (Turner-Walker & Jans Reference Turner-Walker and Jans2008; Hollund et al. Reference Hollund, Jans, Collins, Kars, Joosten and Kars2012). The two waterlogged articulated Bronze Age skeletons from Bradley Fen and Langwell Cist were both free from bioerosion. Waterlogged environments often limit bacterial action but should not prevent putrefactive bone bioerosion completely; the absence of bacterial bioerosion from these samples is therefore unusual (Booth Reference Booth2014). It is possible that these two waterlogged Bronze Age skeletons are those of previously mummified individuals, but the variable effects of waterlogging on putrefaction and bacterial bioerosion mean that this interpretation must remain uncertain (Nielsen-Marsh & Hedges Reference Nielsen-Marsh and Hedges2000; Turner-Walker & Jans Reference Turner-Walker and Jans2008; Hollund et al. Reference Hollund, Jans, Collins, Kars, Joosten and Kars2012).
Distribution of Bronze Age mummified human remains in Britain
The distribution of Bronze Age human skeletal remains demonstrating diagenetic signatures consistent with mummification extends across large areas of Britain (Figure 5; Table 2), regardless of whether disarticulated and waterlogged remains are included; this suggests that mummification was practised throughout Britain during the Bronze Age. These sites are dated to the Early and Late Bronze Age (c. 2200–750 BC), indicating furthermore that mummification was a long-lived mortuary practice. These results raise the question—yet to be addressed—of whether similar funerary treatments were practised more widely among European Bronze Age societies.
Table 2. Catalogue of Bronze Age samples; skeletons that demonstrated histological signatures of mummification are highlighted in bold.
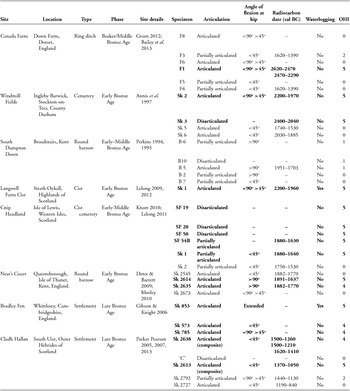

Figure 5. Distribution of Bronze Age sites that included human remains that demonstrated diagenetic signatures consistent with mummification: square = site with articulated ‘mummified’ skeleton(s); circle = site with only disarticulated or partially articulated ‘mummified’ skeleton(s); triangle = site includes a ‘mummified’ skeleton from a waterlogged context.
Methods of Bronze Age mummification
Arrested patterns of bacterial attack were observed within individuals from Neat's Court in Kent (Morley Reference Morley2010) and Bradley Fen in Cambridgeshire (Figure 6; Gibson & Knight Reference Gibson and Knight2006), although mummification techniques may have differed between the two sites. The Neat's Court skeletons demonstrate macroscopic discolouration and fissuring consistent with low-level heat treatment (Figure 7; Deter & Barrett Reference Deter and Barrett2009), suggesting that these bodies may have been mummified by desiccation through smoking. In contrast, the Bradley Fen skeletons display no post-mortem alterations that are indicative of a particular method of mummification; their provenance close to substantial wetlands however, raises the possibility that they were preserved through initial deposition within watery anoxic environments. Bone samples from Windmill Fields in Teesside (Annis et al. Reference Annis, Anderson, Bayliss, Bronk Ramsey, Huntley, Jones, Marshall, Mcgormac, Pearson, Rogers, Rowe, Sedman and Vyner1997), Cnip Headland on the Isle of Lewis, Western Isles of Scotland (Lelong Reference Lelong2011) and Canada Farm in Dorset (Green Reference Green, Meirion Jones, Pollard, Allen and Gardiner2012; Bailey et al. Reference Bailey, Green and Smith2013) were free from bacterial bioerosion, which indicates that bodily putrefaction was curtailed at an early post-mortem stage, and that their treatment may have involved evisceration (Figure 8).

Figure 6. Micrograph of a transverse femoral thin section from Bradley Fen SK 853—an intense band of bacterial bioerosion can be observed a few hundred microns below the periosteal surface, identical to that observed within the Cladh Hallan SK 2638, suggesting that the bone was exposed to limited bodily putrefaction.

Figure 7. SK 2614 from the Neat's Court round barrow on the Isle of Sheppey, Kent, which demonstrated an arrested pattern of bacterial attack consistent with mummification; discolouration of the cranium, teeth and articular ends of long bones suggest that the individual was exposed to low-level burning consistent with artificial preservation by smoking (photograph courtesy of Geoff Morley and Paul Wilkinson).

Figure 8. The primary burial (F1) from the Canada Farm ring ditch; the bone microstructure of this skeleton was perfectly preserved, suggesting that putrefaction was arrested at a very early post-mortem stage, possibly by evisceration (photograph courtesy of Martin Green).
The evidence for variability in methods of mummification is consistent with suggestions that Bronze Age communities made innovative use of available local resources to preserve their dead (Parker Pearson et al. Reference Parker Pearson, Chamberlain, Craig, Marshall, Mulville, Smith, Chenery, Collins, Cook, Craig, Evans, Hiller, Montgomery, Schwenninger, Taylor and Wess2005). Techniques that produced a partial or ephemeral mummy might have been deliberately used by British Bronze Age communities to enable fragmentation, circulation and recombination of bodies and anatomical parts. Consistent production of such relatively short-lived mummies might partly explain why preserved soft tissue of Bronze Age individuals has not usually survived archaeologically (with the exception of some bog bodies); Britain's temperate climate is generally poorly suited for long-term soft tissue preservation above or below ground in any case.
The Cladh Hallan bodies had been manipulated into tightly flexed positions (leg flexion at the hip was above 45ᵒ), suggesting that they may have been wrapped (Parker Pearson et al. Reference Parker Pearson, Chamberlain, Craig, Marshall, Mulville, Smith, Chenery, Collins, Cook, Craig, Evans, Hiller, Montgomery, Schwenninger, Taylor and Wess2005). Body position was highly variable amongst the Bronze Age mummified skeletons identified here and there is no significant association between posture and OHI score (n = 27, Kruskal–Wallis X2 = 3.50453, p = 0.3202). There is no regional variation in posture amongst the mummified specimens and positions often varied considerably across single sites (Table 2). Evidence for tight wrapping of bodies in the Bronze Age does not equate to mummification, although prior mummification may provide an explanation for articulated skeletons that appear to have been manipulated beyond what might be possible on a fresh corpse (Parker Pearson et al. Reference Parker Pearson, Chamberlain, Craig, Marshall, Mulville, Smith, Chenery, Collins, Cook, Craig, Evans, Hiller, Montgomery, Schwenninger, Taylor and Wess2005).
Conclusion
Microscopic analysis of diagenesis in a dataset of 307 samples of human bone recovered from 26 archaeological sites in Europe reveals that 16 of those 34 British human remains dating to the Bronze Age (c. 2200–750 BC) demonstrate an unusual pattern of arrested bacterial bioerosion. These same patterns of histological preservation have been observed regularly within bone samples from mummified individuals. The Bronze Age assemblage includes samples of skeletons retrieved from the Cladh Hallan settlement where there is a suite of evidence that at least two (composite) bodies had formerly been mummified (Parker Pearson et al. Reference Parker Pearson, Chamberlain, Craig, Marshall, Mulville, Smith, Chenery, Collins, Cook, Craig, Evans, Hiller, Montgomery, Schwenninger, Taylor and Wess2005, Reference Parker Pearson, Chamberlain, Collins, Cox, Craig, Craig, Hiller, Marshall, Mulville and Smith2007, Reference Parker Pearson, Cox Willis, Marshall, Mulville, Smith, Cowie, Craig, Deluis, Juddery, Manley, Schwenninger and Taylor2013).
The simplest explanation for the persistence of these diagenetic signatures is that Bronze Age populations throughout Britain practised mummification on a proportion of their dead. The numbers of disarticulated bone samples that display the diagenetic signature of prior mummification and the occasional evidence for deliberate reconstruction of anatomical parts suggest that a significant proportion of buried Bronze Age mummies may be composites. The common appearance of diagenetic signatures of mummification on Bronze Age bone samples might lead us to infer that this practice was introduced as one aspect of the cultural changes associated with the appearance of metalworking and other Bronze Age innovations in, for example, ceramic or textile manufacture.
Perhaps more plausible is the probable growing role of deceased ancestors in the legitimation of rights over land and property. Increasing concerns with the genealogical significance of individual ancestors are evident in the round-barrow cemeteries of the earlier Bronze Age (c. 2200–1500 BC; e.g. Garwood Reference Garwood and Last2007). The second millennium BC in Britain was associated with increasing pressures on land use and intensification of agriculture (Field Reference Field2008: 71–83), especially from 1600–1500 BC onwards, as is evident in the laying out of co-axial field systems (e.g. Yates Reference Yates2007).
Whatever the motives were for adopting practices of post-mortem preservation, these results confirm the value of microscopic examination of bone microstructure. Indeed, it may be the only consistent method for identifying formerly mummified skeletons in the archaeological record. Further research is required to confirm the extent and nature of these practices in later prehistoric Britain, and whether they extended into continental Europe. One line of inquiry could involve investigating skeletons from Bronze Age sites that demonstrate anomalous early radiocarbon dates, although the success of this approach would depend upon the precision of dating methods and the interval between death and burial.
Acknowledgements
This research formed part of an Arts and Humanities Research Council doctoral studentship undertaken at the University of Sheffield. We would like to thank the following people for granting access to sample remains: Eamonn Kelly and Isabella Mulhall (National Museum of Ireland), Don Brothwell (University of York), Geoff Morley (MOLES Archaeology), Paul Wilkinson (Swales and Thames Archaeological Survey Company), Olivia Lelong (Northlight Heritage), Mark Knight (Cambridge Archaeological Unit), Peter Rowe (Tees Archaeology), Martin Smith (University of Bournemouth) and Martin Green.